Heat conduction and its related interdisciplinary areas
从燧人氏钻木取火开始, 人类文明的发展就和“热能”息息相关, 对热现象的认识贯穿整个人类社会发展的历程. 全球约 60%的能源都以废热的形式白白浪费. 热物理的进展对于我国最近强调的 “碳达峰、碳中和”至关重要. 我国力争于 2030年前二氧化碳排放达到峰值, 2060年前实现碳中和.而在利用的和废弃的能源中, 占比最大的都是热能. 关于热能的研究涉及材料、电子器件、生物、能源等诸多学科, 纳米工程的引入为热物理的进展注入了新的活力. 微纳米电子器件、电池中的过热问题会直接影响其性能和使用寿命, 成为 5G、量子计算、芯片、电动汽车等国家战略技术领域目前的研究热点. 人类对于纳米尺度和跨尺度中热物理的认知还是远远不够的. 因此, 物理、工程、材料、电子等多学科的交叉研究将在上述新兴领域大有用武之地.
鉴于热传导问题研究的挑战性与紧迫性, 受编辑部委托特组织本专题, 报道热传导领域最新进展和前沿态势. 陈亮、冯芒老师综述了基于离子阱中离子晶体的热传导的研究进展, 包括一维、二维和三维模型中温度分布和稳态热流的计算方法, 还讨论了无序度对离子晶体热导性的影响; 鲍华老师回顾了金属导热研究的历史, 并对最近十几年来金属导热的研究进行了总结, 特别是对基于第一原理电子-声子耦合模式分析的金属导热机理的研究进行了综述; 唐桂华老师基于宏观热流调控的思想, 提出了非封闭式热斗篷, 并用于高超声速飞行器头锥热防护. 希望本专题对读者了解此前沿领域有所帮助, 为热传导及其相关交叉领域领域的学术交流做一点贡献.
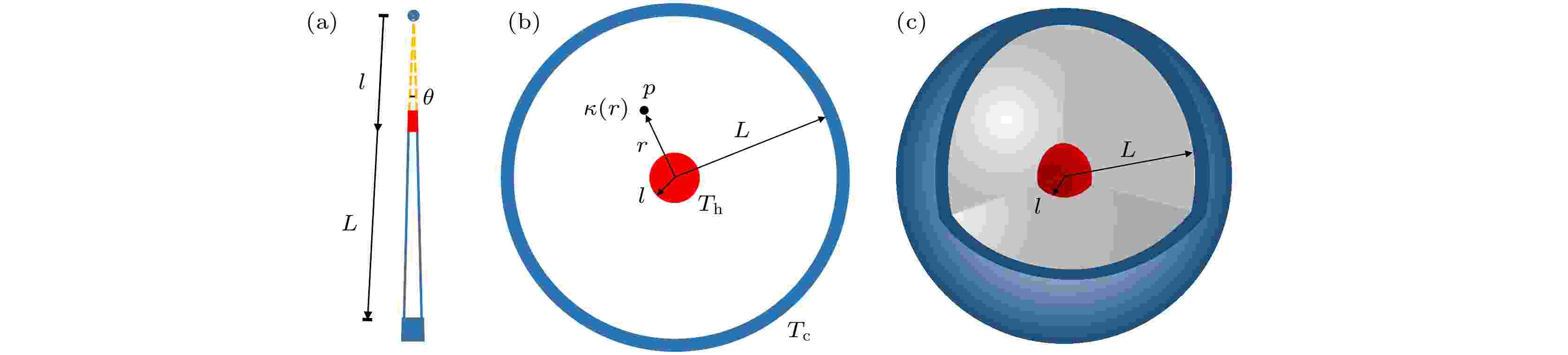
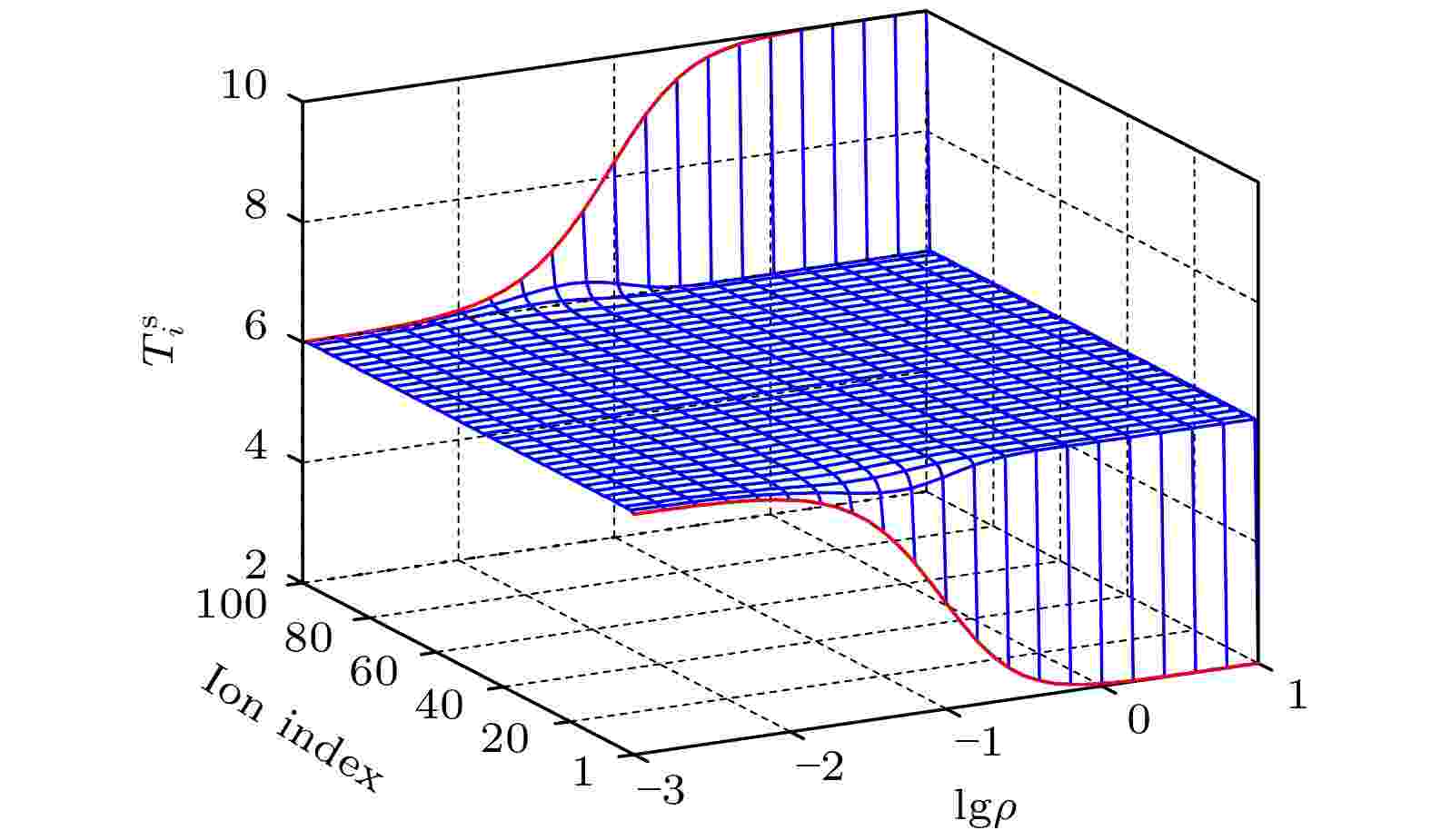
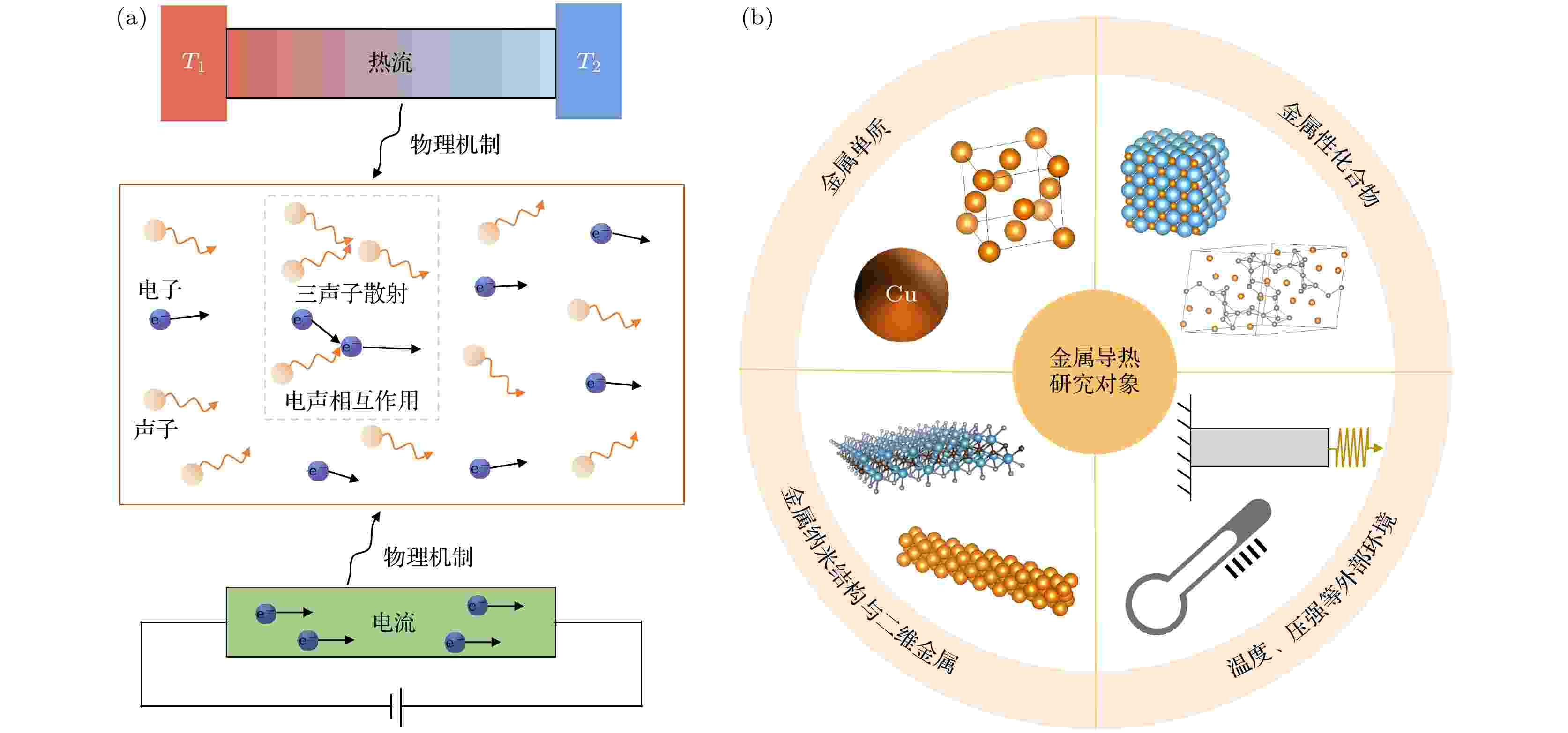
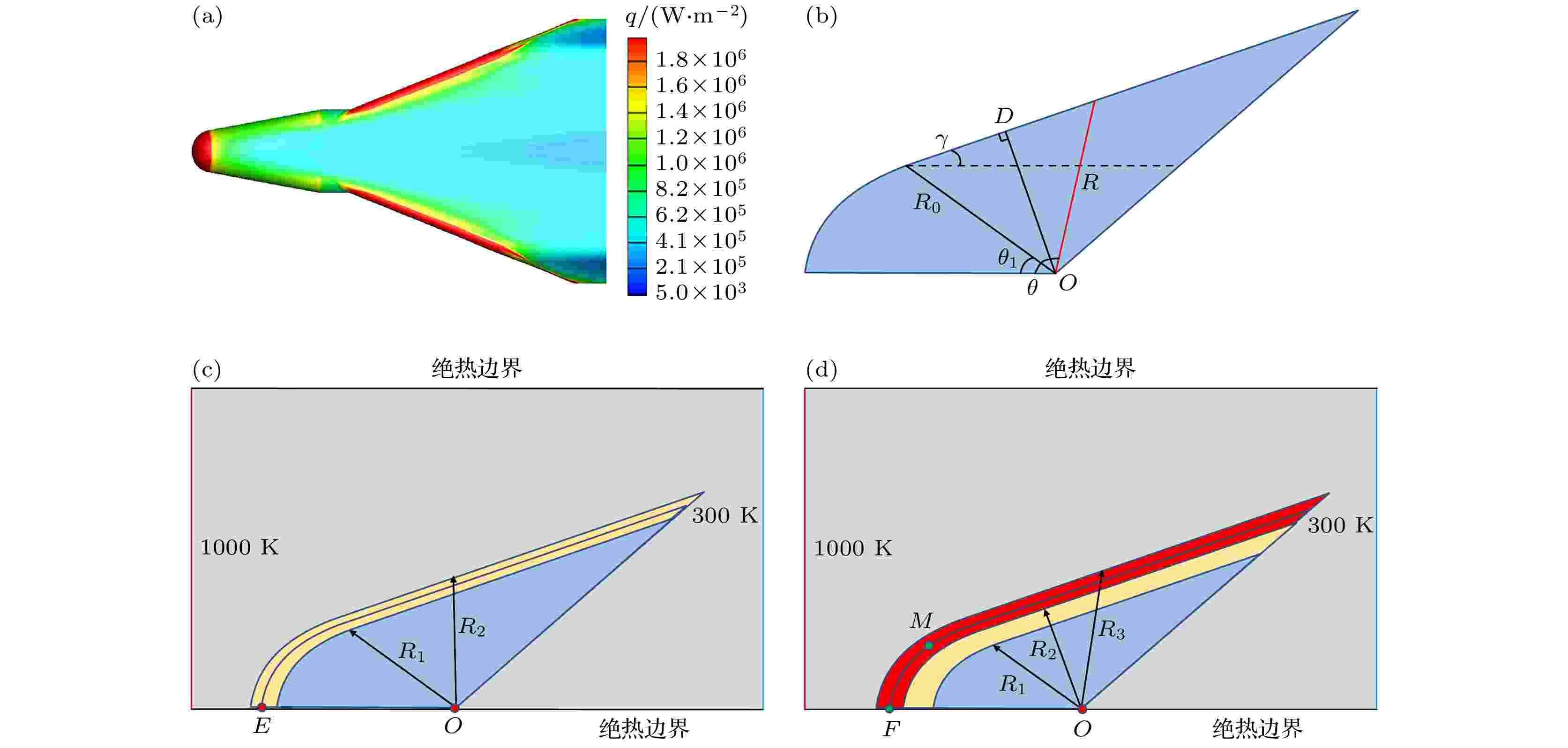