Highlights
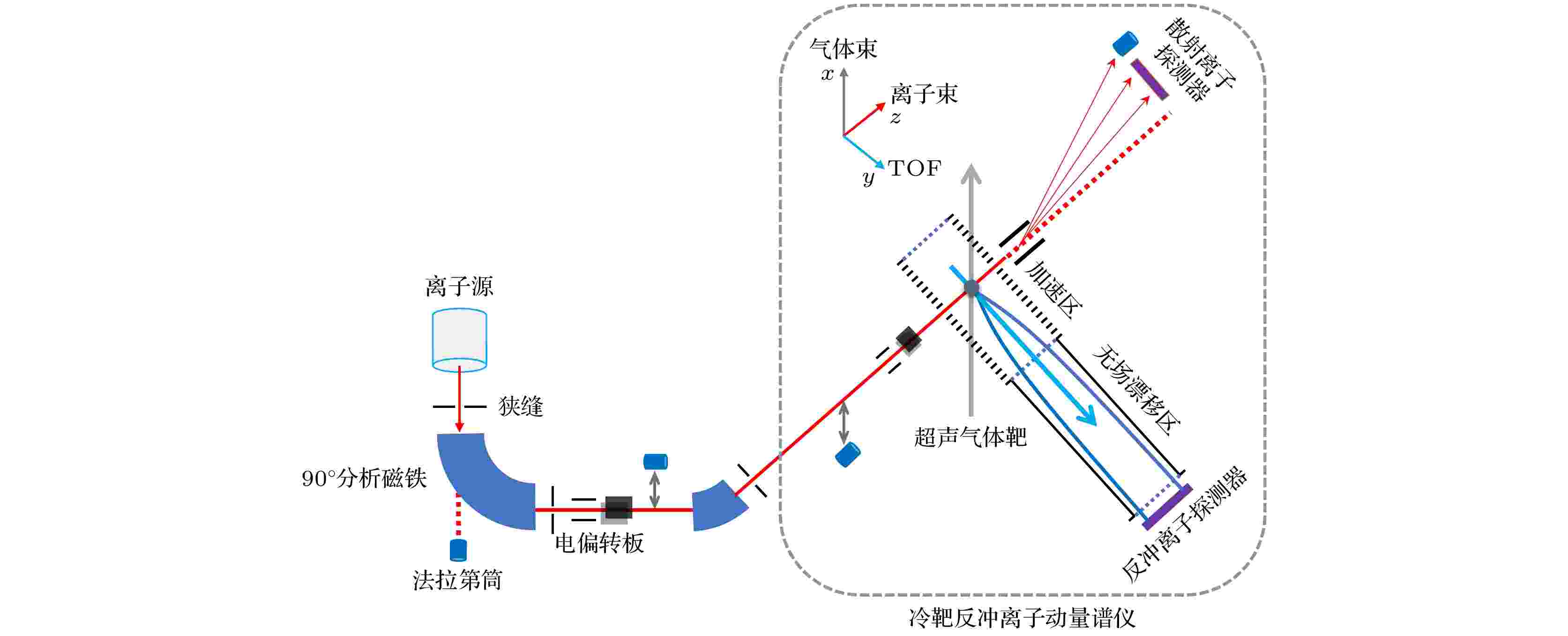
SPECIAL TOPIC—Dynamics of atoms and molecules at extremes
COVER ARTICLE
2024, 73 (24): 240701.
doi: 10.7498/aps.73.20241290
Abstract +
Electron capture in the collision of highly charged ions with atoms and molecules is a fundamental process related to the electron transition between bound states belonging to two atomic-centers. The X-ray emission after electron capture is important for X-ray astrophysical modeling, fusion plasma diagnostics, and ion irradiated biophysics. In the past few decades, momentum-imaging cold-target recoil ion momentum spectroscopy has been a significantly developed technique and widely used to measure the quantum state-selective population in electron capture processes. Based on the cold target recoil ion momentum spectroscopy installed on the 150 kV highly charged ion platform in Fudan University, Shanghai City, China, the state-selectivity of double electron capture in the bombardment of 1.4–20 keV/u Ar8+ on He is measured, and the relative cross sections of the 3l 3l' to 3l 7l' double excited states are obtained. It is found that with the increase of collision energy, more quantum state-selectivity channels are open in the double electron capture of Ar8+-He collision. It is also found that the relative cross section of the quantum state population is strongly dependent on the collision energy of the projectile ion. The present measurements not only enrich the state-selective cross-sectional library and collision dynamics of highly charged ion charge exchange processes, but also provide experimental benchmarks for existing theoretical calculations.
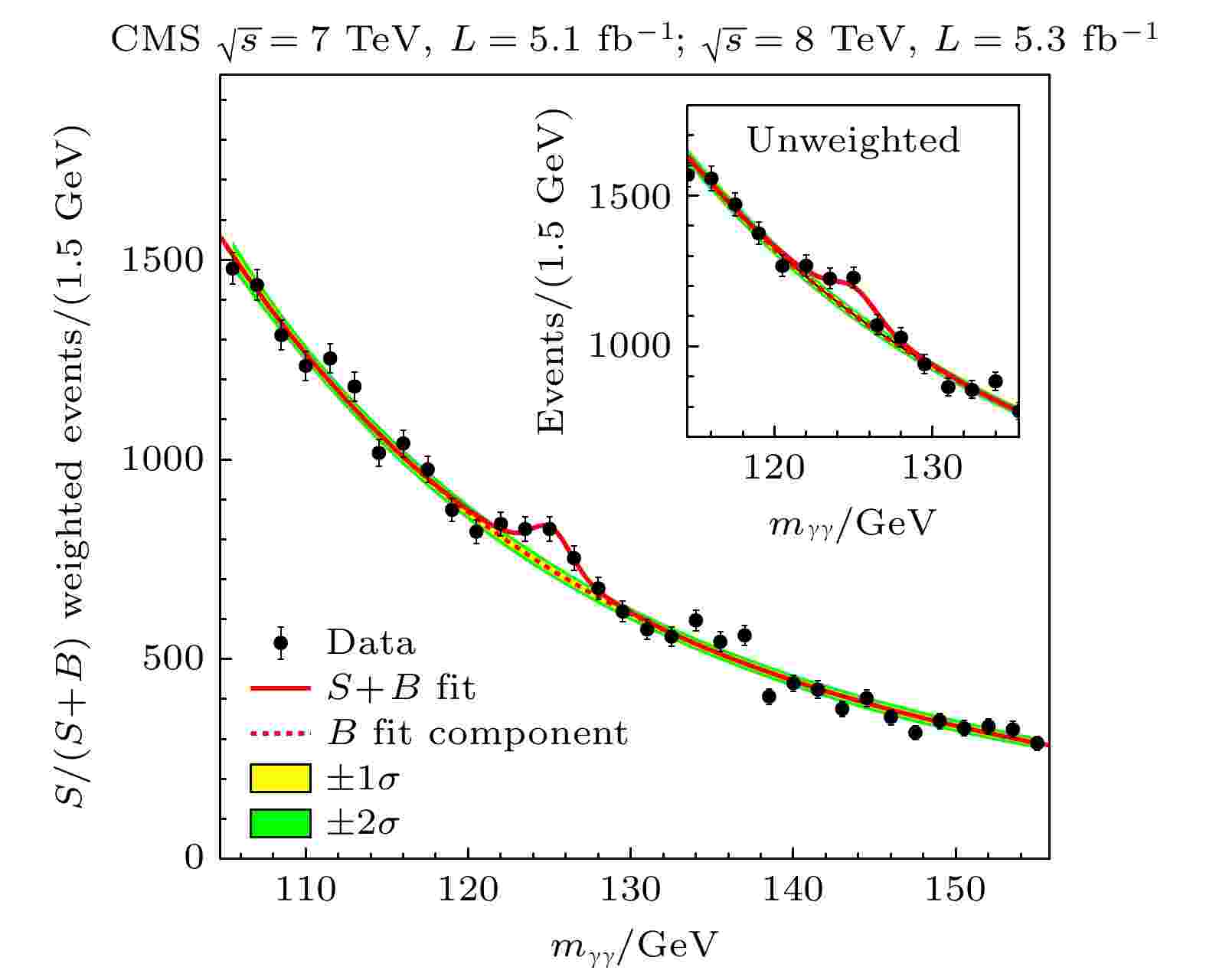
Abstract +
This article reviews the discovery of the Higgs boson, discusses the studies of its properties, and introduces the physical prospects of the future Higgs factories. The greatest goal of particle physics is to understand the fundamental particles of the universe and how they interact with each other (or more generally, how the universe operates). In the standard model of particle phyiscs, the Higgs mechanism is proposed to explain the origin of elementary particle mass and predict the existence of the Higgs boson. Higgs physics is one of the most important research areas in particle physics. The Large Hadron Collider (LHC) at CERN (Geneva, Switzerland) accelerates proton beams to collide at center-of-mass energy of 13 TeV, thus defining the world’s energy frontier. The ATLAS and CMS detectors are two general-purpose detectors at the LHC for studying the debris from the collisions. The Higgs boson was discovered in the ATLAS and CMS experiments in 2012. This discovery completed the fundamental particle spectrum of the standard model and was an important milestone for particle physics. Since then, many studies have been conducted on the properties of Higgs boson, including spin, mass and couplings, to deepen our understanding of the Higgs mechanism. In particular, the Higgs boson couplings to fermions and to themselves present new kinds of fundamental interactions with paramount significance, which have not been fully confirmed. Additionally, the Higgs bosons has become an important tool to search for dark matter, heavy resonance, and other new physical phenomena. So far, there has been no deviation from the predictions of the standard model. Looking forward to the future, it is proposed to use the electron-positron collisions to study the Higgs boson in more depth. Physics studies have shown that these Higgs factories can significantly improve the accuracy of many properties of the Higgs boson, including width and couplings, and provide great physics prospects.
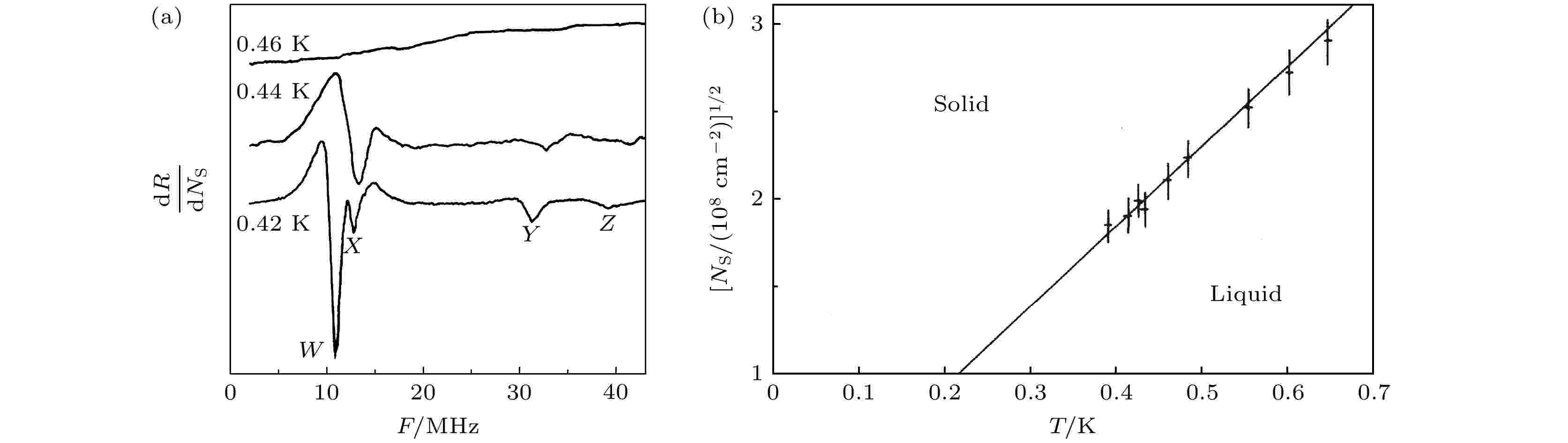
Abstract +
In 1934, Eugene Wigner, who was studying at Princeton University, predicted the existence of electronic crystals. Electrons have both kinetic energy and potential energy of interaction. When the density of electronic states satisfies certain conditions, due to the repulsion between electrons, electrons will tend to arrange themselves in a regular lattice structure, forming electron crystals, which is also known as Wigner crystals. For nearly 90 years, Wigner crystals have fascinated condensed matter physicists. Physicists have designed many ingenious semiconductor heterojunctions to obtain lower electron densities and added magnetic fields to achieve larger effective mass of electron. In 1979, experiments revealed the existence of a phase transition from an electron liquid phase to an electron crystal on the surface of liquid helium, and subsequent experiments observed the characteristics of two-dimensional (2D) Wigner crystals in 2D electron gas under high magnetic fields. However, direct observation of 2D Wigner lattices in real space remains a formidable challenge. Through the graphene sensing layer of WSe2/WS2 moiré superlattice, Hongyuan Li, Feng Wang, et al. observed the real-space morphologies of Wigner crystals in their experiments. And in a recent study, researchers used high-resolution scanning tunneling microscopy to directly image magnetic field-induced Wigner crystals in Bernal stacking bilayer graphene and investigated their structural properties as a function of electron density, magnetic field, and temperature. In this paper, we will introduce some interesting things about Wigner crystals through four representative researches briefly.
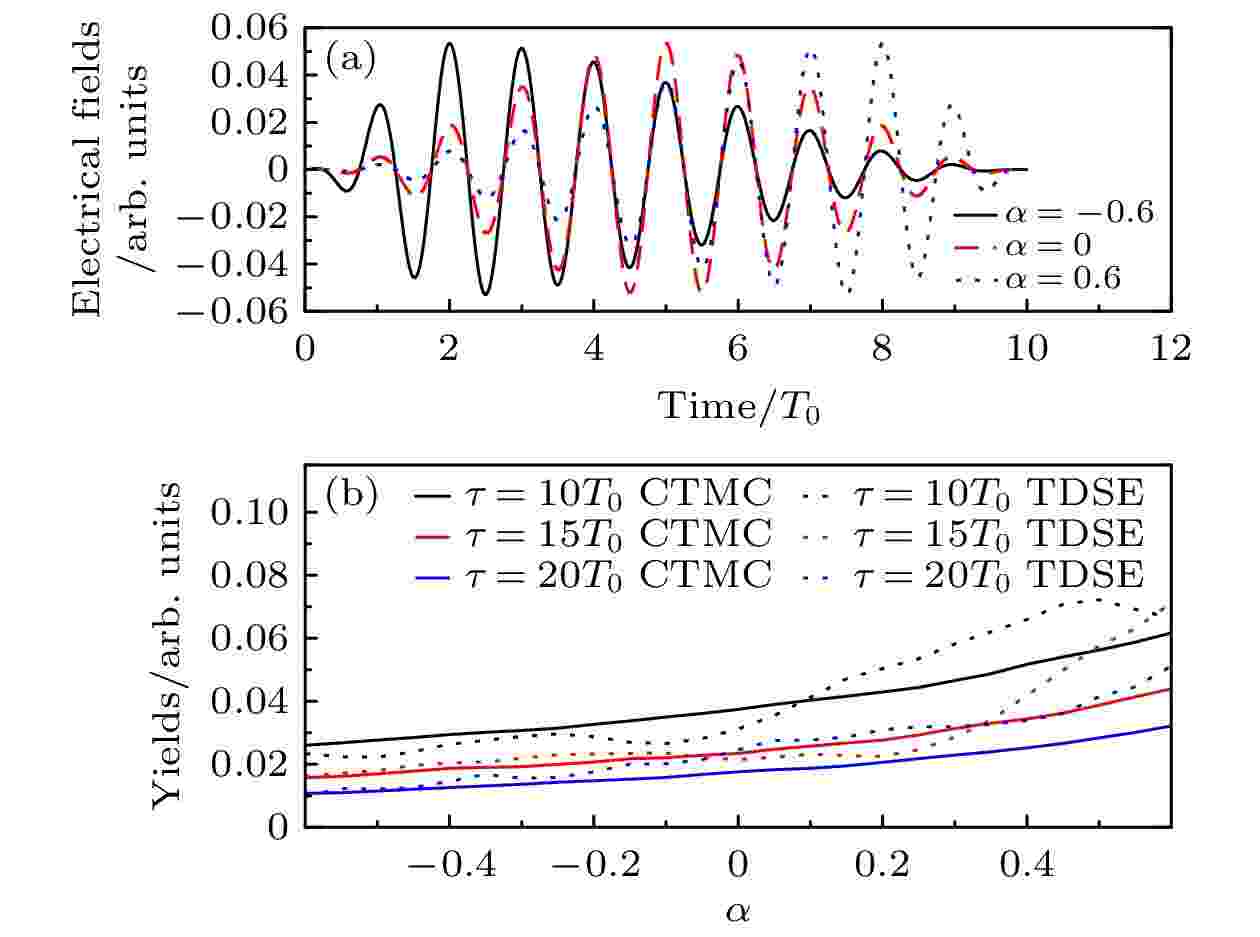
SPECIAL TOPIC—Dynamics of atoms and molecules at extremes
EDITOR'S SUGGESTION
2024, 73 (24): 243201.
doi: 10.7498/aps.73.20241222
Abstract +
Rydberg atoms are important building blocks for quantum technologies, and because of their unique tunable quantum properties, they possess new applications in quantum computing, quantum communication, and quantum sensing. Besides the widely-used few-photon resonant excitation for the specific Rydberg state, multiple Rydberg states can be populated coherently and efficiently through the frustrated tunneling ionization or the Coulomb potential recapture effect in a strong laser field. The excitation of Rydberg states in a strong field provides an opportunity for realizing the ultrafast quantum control on Rydberg atom and bridging the gap between strong field physics and quantum information technology. Using the classical trajectory Monte Carlo method and Qprop package to solve time-dependent Schrödinger equation, we calculate the population of Rydberg states. Our results show that the population increases with the increase of parameter of the asymmetric laser envelope. Based on the quantitative rescattering theory, the calculated time-dependent recapture rate is negatively related to the laser envelope and the residual laser interaction time, which is termed the envelope effect. Combined with the carrier-wave effect, an analytic formula can be used to calculate the Rydberg state population: $ Y(t) \propto $$ W_0\left(t\right) \dfrac{t-\tau+c}{f\left(t\right)} \cos \left(\omega t+\phi\right) . $ This result opens the way to enhancing the generation of Rydberg states by using the laser envelope control, which is beneficial to the future quantum technology based on the Rydberg states generated in the strong laser field.
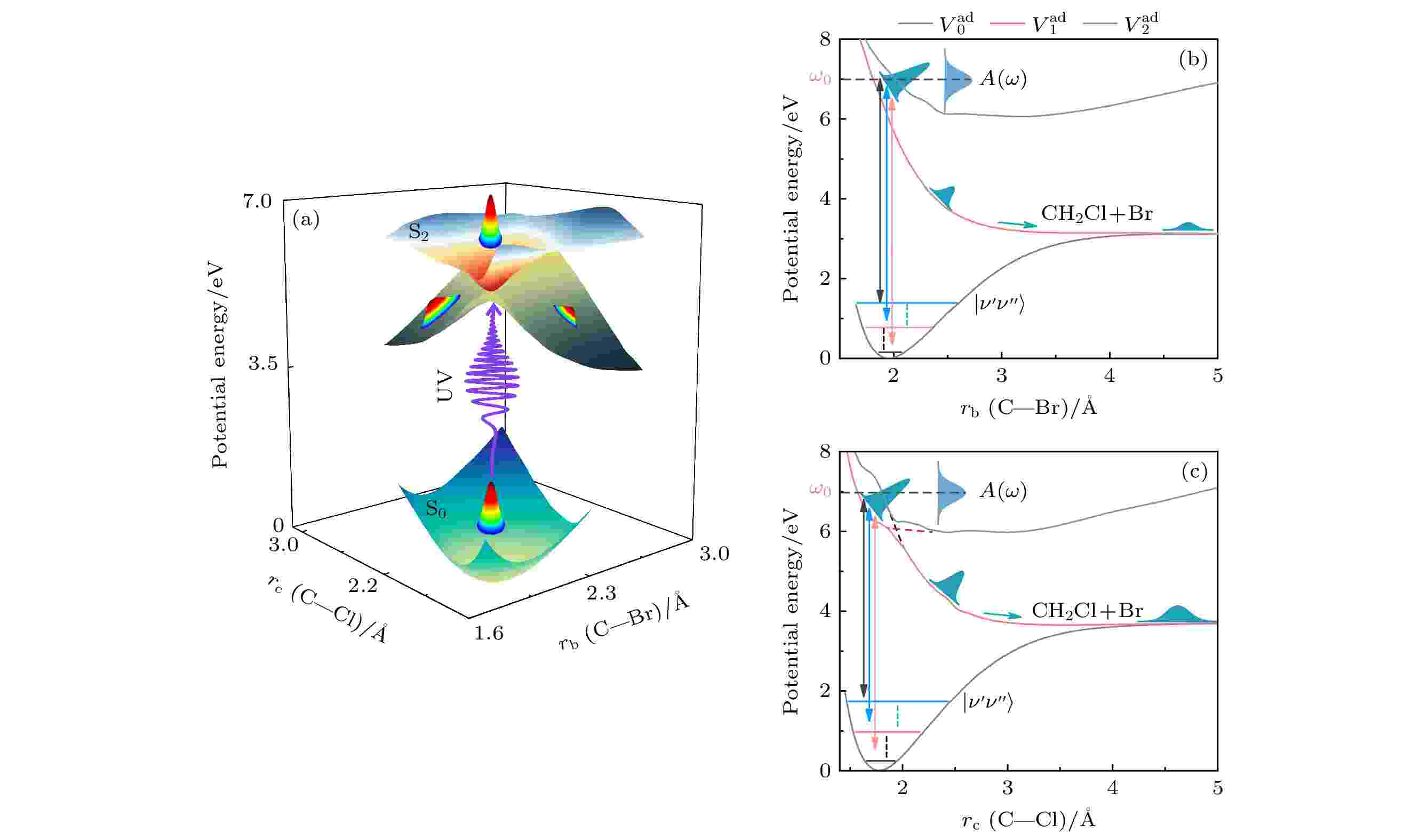
SPECIAL TOPIC—Dynamics of atoms and molecules at extremes
EDITOR'S SUGGESTION
2024, 73 (24): 243301.
doi: 10.7498/aps.73.20241401
Abstract +
Coherent control of molecular dissociation in ultrafast strong fields has received considerable attention in various scientific disciplines, such as atomic and molecular physics, physical chemistry, and quantum control. Many fundamental issues still exist regarding the understanding of phenomena, exploration of mechanisms, and development of control strategies. Recent progress has shown that manipulating the spectral phase distribution of a single ultrafast strong ultraviolet laser pulse while maintaining the same spectral amplitude distribution can effectively control the total dissociation probability and branching ratio of molecules initially in the ground state. In this work, the spectral phase control of the photodissociation reaction of chlorobromomethane (CH2BrCl) is studied in depth by using a time-dependent quantum wave packet method, focusing on the influence of the initial vibrational state on the dissociation reaction. The results show that modifying the spectral phase of a single ultrafast pulse does not influence the total dissociation probability or branching ratio in the weak field limit. However, these factors exhibit significant dependence on the spectral phase of the single ultrafast pulse in the strong field limit. By analyzing the population distribution of vibrational states in the ground electronic state, we observe that chirped pulses can effectively control the resonance Raman scattering (RRS) phenomenon induced in strong fields, thereby selectively affecting the dissociation probability and branching ratio based on initial vibrational states. Furthermore, we demonstrate that by selecting an appropriate initial vibration state and controlling both the value and sign of the chirp rate, it is possible to achieve preferential cleavage of Cl+CH2Br bonds. This study provides new insights into understanding of ultrafast coherent control of photodissociation reactions in polyatomic molecules.
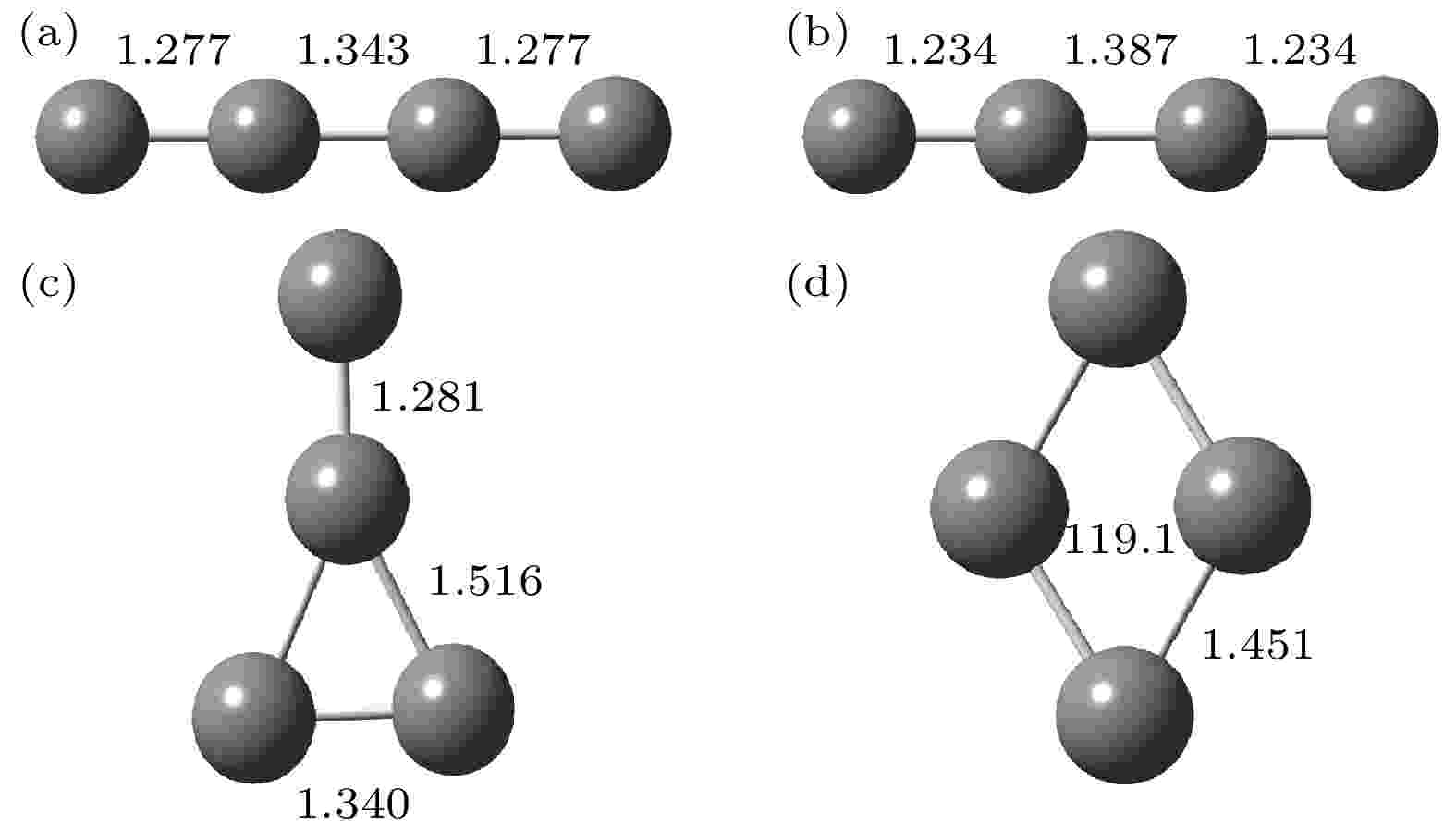
SPECIAL TOPIC—Dynamics of atoms and molecules at extremes
EDITOR'S SUGGESTION
2024, 73 (24): 243401.
doi: 10.7498/aps.73.20241377
Abstract +
This paper reports low-energy electron scattering with $ {{\mathrm{C}}}_{4}^{-} $ anions by using the ab initio R -matrix method in the single state close-coupling (CC) model and the fixed-nuclei approximation. We predict the elastic integral scattering cross sections (ICSs) of four conformers of $ {{\mathrm{C}}}_{4}^{-} $ ions in an energy range of 0 < E ≤12 eV and discuss the effects of configuration changes on resonance position and width. Additionally, the theoretical results and experimental data are compared and analyzed. The results indicate that the 8.8 eV resonance peak observed in experiment is mainly derived from the $ {{{\Sigma }}}_{{\mathrm{u}}}^{+} $ and $ {{{\Sigma }}}_{{\mathrm{u}}}^{-} $ resonances of the conformer A and the A2 resonance of the conformer C. The scattering cross-section reveals that the conformer A has five resonant states, and the conformer B has three resonances, while C and D each have four resonances. Finally, we use the Boltzmann distribution to calculate the populations of different conformers at different temperatures, and simulate the low-energy electron elastic integrated scattering cross-section at room temperature, which is in good agreement with available experimental results. We also find a shape resonance with a width of 0.20 eV at 3.3 eV in our total cross sections, which is not detected in the existing experimental results. This provides new opportunities for measurement.
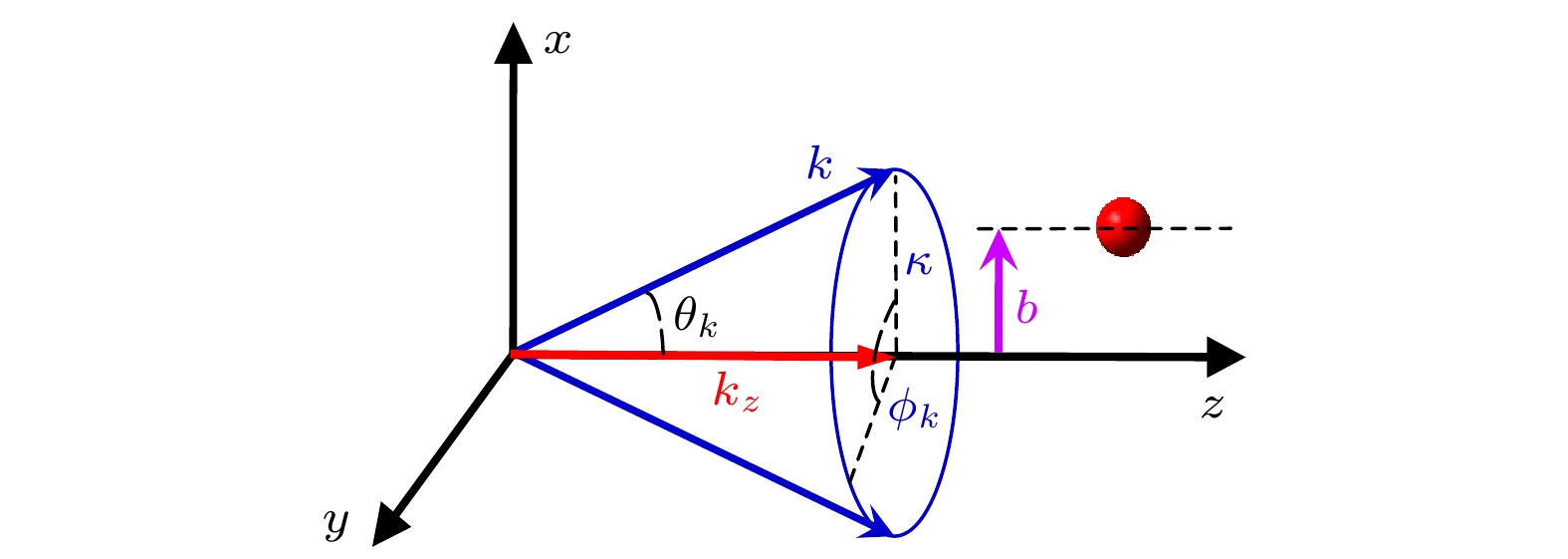
SPECIAL TOPIC—Dynamics of atoms and molecules at extremes
EDITOR'S SUGGESTION
2024, 73 (24): 244201.
doi: 10.7498/aps.73.20241378
Abstract +
Owing to vortex light possessing the additional orbital angular momentum, its interaction with atoms and molecules can reveal in more depth insights into dynamics than the plane wave light. This paper aims to establish a theoretical framework for the photoionization of atoms and molecules by Bessel vortex light. In the case of macroscopic gas target, helium atoms are randomly dispersed around the entire region of the Bessel vortex beam. The final photoionization cross-section is not dependent on the angular momentum of the vortex light, but depends on the opening angle of the Bessel vortex light. This paper systematically computes the variation of photoionization cross-section with photon energy and the angular distributions of photoelectrons under different geometric conditions. The computation results demonstrate that there is a significant difference in the photo-ionization cross-section between vortex light and plane wave light. In order to further investigate the characteristics of the phase singularity of the vortex light (when the light intensity reaches zero), this paper further calculates the photo-ionization of the vortex light with opening angles of 5°, 30°, and 60° at the phase singularity, respectively. The results indicate that the angular distribution of photoelectrons at these three angles is significantly dependent on the orbital angular momentum and the opening angle of the vortex light, and the calculated absolute cross-section does not equate to zero. This represents an important distinguishing feature of the Bessel vortex light when interacting with atoms, distinguishing it from the plane wave. This work lays the foundation for further studying vortex light photo-ionization and their applications.
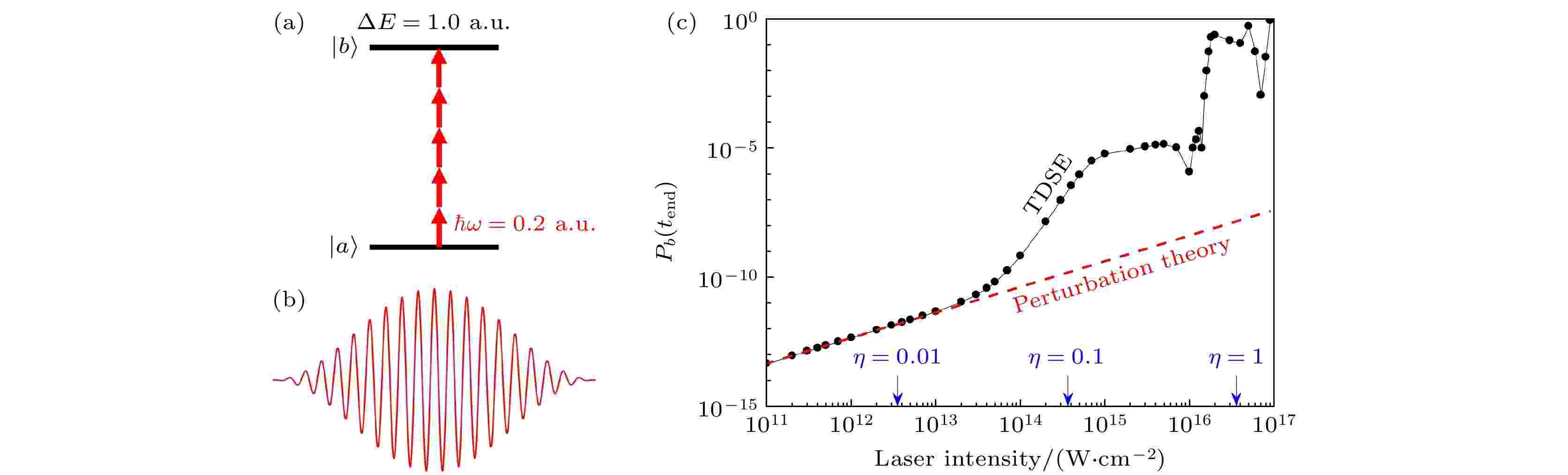
SPECIAL TOPIC—Dynamics of atoms and molecules at extremes
EDITOR'S SUGGESTION
2024, 73 (24): 244202.
doi: 10.7498/aps.73.20241456
Abstract +
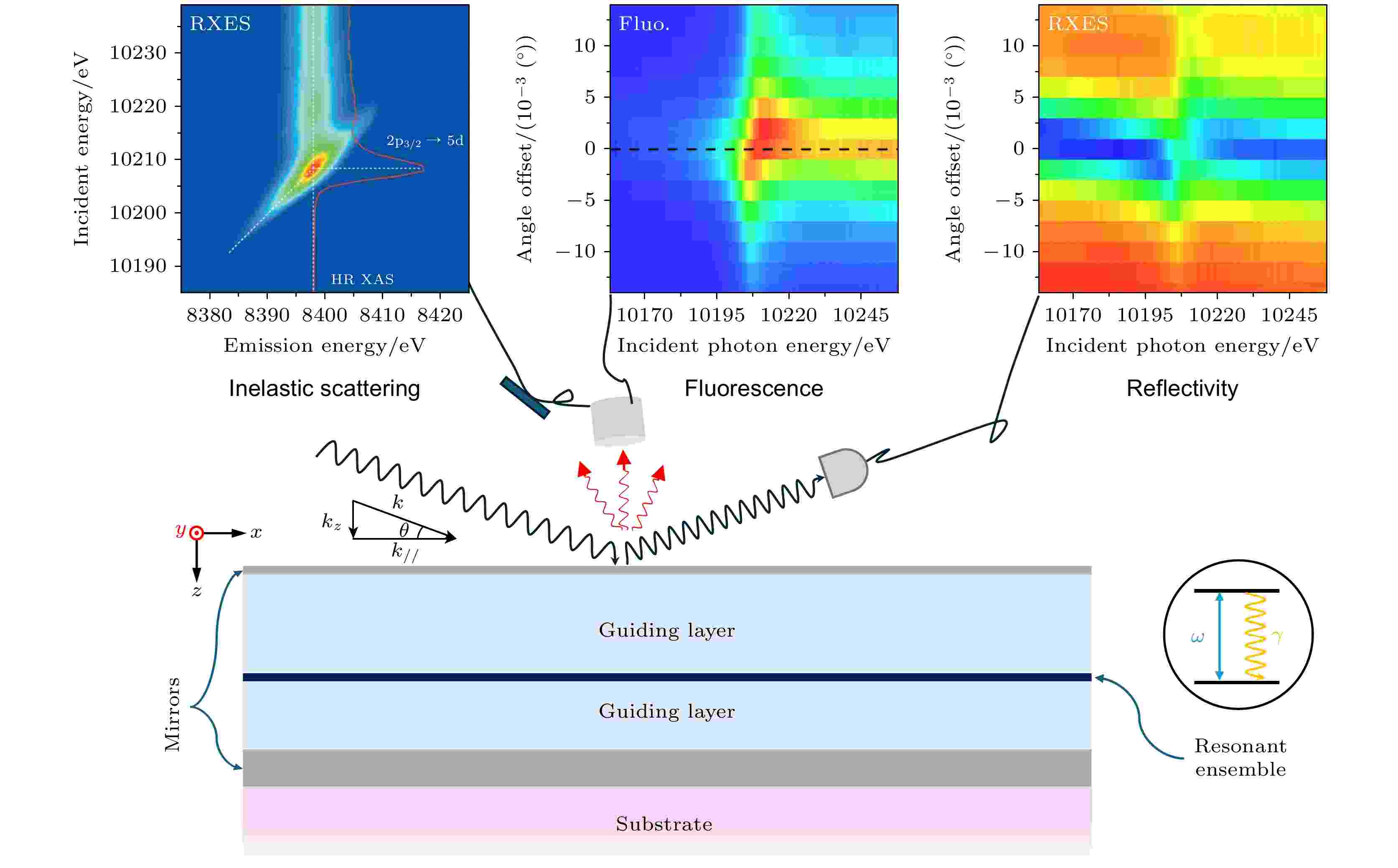
SPECIAL TOPIC—Dynamics of atoms and molecules at extremes
EDITOR'S SUGGESTION
2024, 73 (24): 246101.
doi: 10.7498/aps.73.20241218
Abstract +
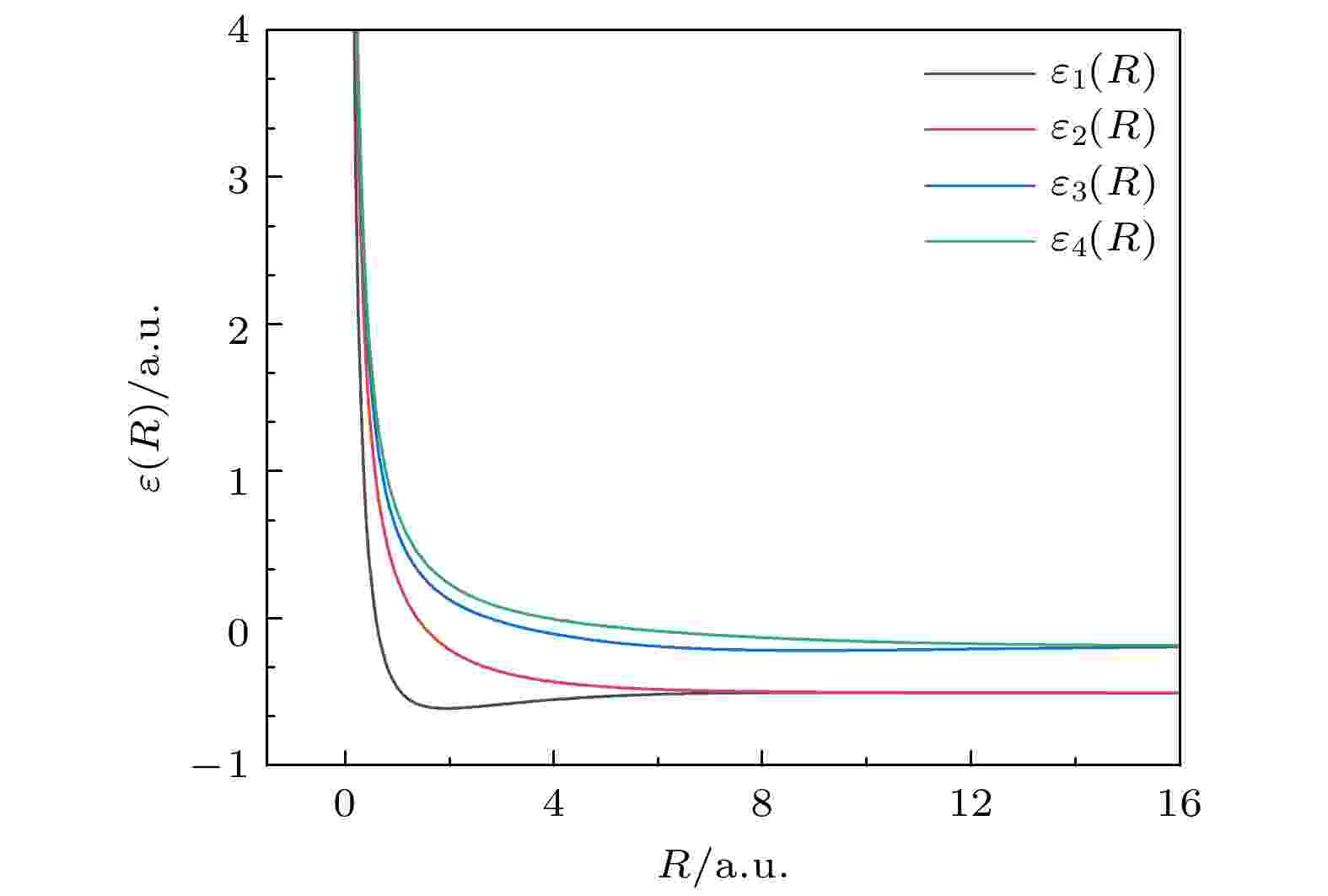
SPECIAL TOPIC—Dynamics of atoms and molecules at extremes
EDITOR'S SUGGESTION
2024, 73 (24): 248201.
doi: 10.7498/aps.73.20241283
Abstract +
In recent years, the rapid development of ultrashort pulse laser technology has made it possible to regulate the ionization and dissociation dynamics of atoms and molecules. Among them, the microscopic dynamics of molecular dissociation have always been a hot topic. The phenomenon of molecular dissociation, which is caused by the interaction between femtosecond intense laser fields and $\text{H}_2^+$ molecules, has attracted widespread attention. Previous theoretical studies on the dissociation of $\text{H}_2^+$ molecules mainly focused on studying its dissociation dynamics through numerical calculations, with relatively few theoretical models. This paper aims to establish a simple classical model to describe the dissociation dynamics. Firstly, this paper calculates the joint distribution of nuclear energy and electronic energy in the dissociation process of $\text{H}_2^+$ molecules under the action of pump lasers by numerically solving the Schrödinger equation. The results prove that $\text{H}_2^+$ molecules initially in the ground state are dissociated into ${\rm H}^+ + {\rm H}^*$ after absorbing a pump photon in the pump light field. Next, this paper studies the dissociation dynamics of $\text{H}_2^+$ molecules in time-delayed two-color femtosecond lasers. We find that it greatly depends on the specific forms of the pump light and the probe light. By utilizing the dependence of the dissociation kinetic energy release (KER) spectrum on the time delay of the two-color femtosecond lasers, we retrieve the sub-attosecond microscopic dynamic behaviors of electrons and atomic nuclei in the dissociation process. Furthermore, we establish a classical model based on the conservation of energy and momentum to describe the dissociation dynamics. This model can qualitatively predict the ion dissociation KER spectrum depending on the time delay of the two-color femtosecond lasers. The electronic resonant transition between the molecular ground state and the first excited state caused by the probe light will affect the ion kinetic energy spectrum in the dissociation process. Namely, the ion kinetic energy spectrum is dependent on the frequency of the probe laser. By taking advantage of this characteristic, we propose a scheme to reconstruct the evolution of the internuclear distance with time. Our reconstruction results can qualitatively predict the trend of the numerical simulation results, and this scheme may provide some theoretical guidance for experiments.
- 1
- 2
- 3
- 4
- 5
- ...
- 179
- 180