Vol. 74, No. 10 (2025)
2025-05-20
GENERAL
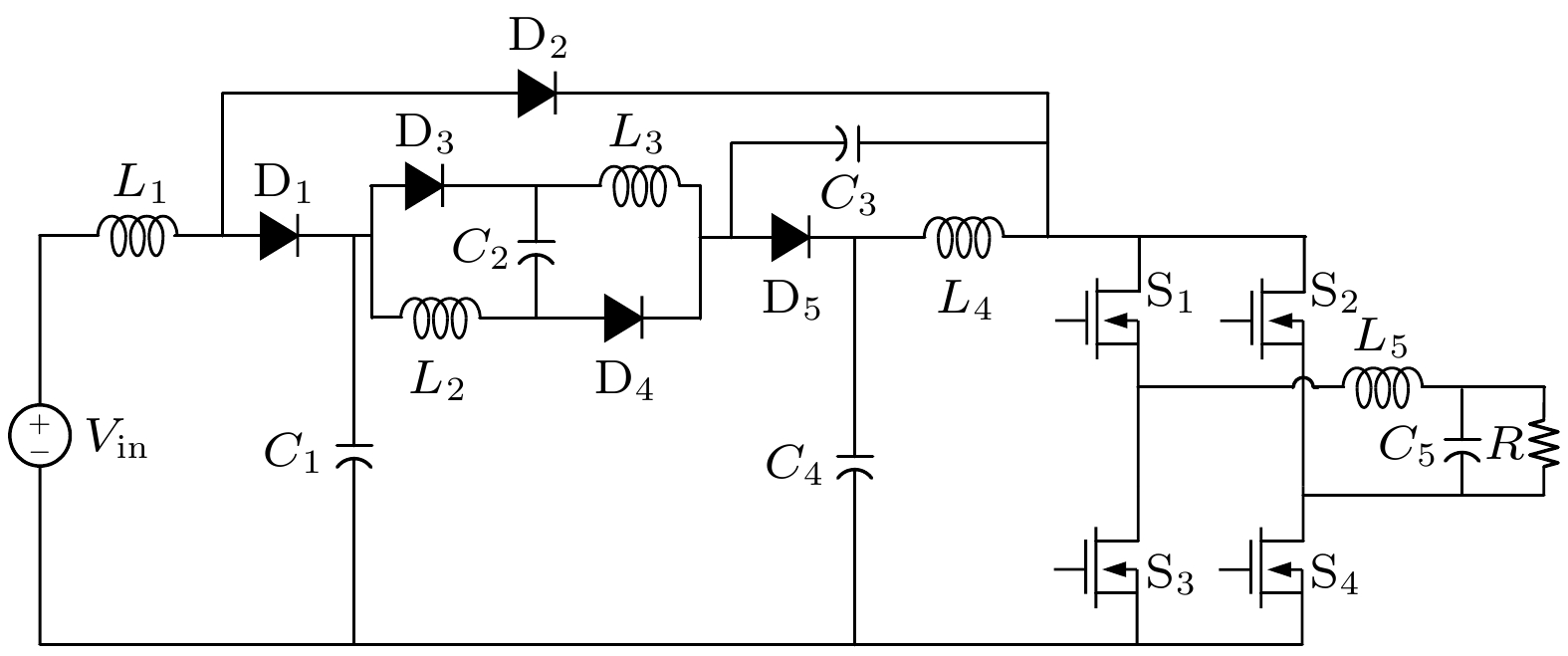
2025, 74 (10): 100201.
doi: 10.7498/aps.74.20241798
Abstract +
Microinverters have been widely used in distributed photovoltaic (PV) systems in recent years due to their modularity and flexibility. However, the current development of microinverter topologies faces significant challenges, such as low voltage gain and limited reliability. To solve these problems, an enhanced switched-inductor quasi-Z-Source inverter (ESL-qZSI) based on gallium nitride high electron mobility transistor (GaN HEMT) is proposed in this work. The proposed inverter introduces a novel topology that integrates an auxiliary boost unit with a switched-inductor quasi-Z-source network. This topology significantly enhances the voltage gain at low shoot-through duty ratios and reduces the voltage stress across the switching device. Additionally, the use of GaN HEMT as power switching components increases the switching frequency from the traditional 10 kHz to 100 kHz, in which a specialized negative turn-off gate driver circuit is designed to adapt the characteristics of the GaN HEMT and to ensure reliable switching operation. This increase in frequency reduces the size of passive components, such as inductors. Experimental results show that the proposed inverter achieves a boost factor of 5.75 at a shoot-through duty ratio of 0.2, which indicates that its performance is improved by 15% and 91% greater than the traditional switched-inductor-capacitor quasi-Z-source inverter (SLC-qZSI) and the traditional switched-inductor Z-source inverter (SL-ZSI), respectively. These results confirm that the proposed inverter enhances the voltage gain of existing topologies. Besides, compared with SLC-qZSI, the proposed inverter can obtain a higher efficiency of 90.5%, which shows the advantage of efficiency. In conclusion, the proposed ESL-qZSI with GaN HEMT provides a hopeful solution for high-efficiency and compact microinverter systems in photovoltaic applications.
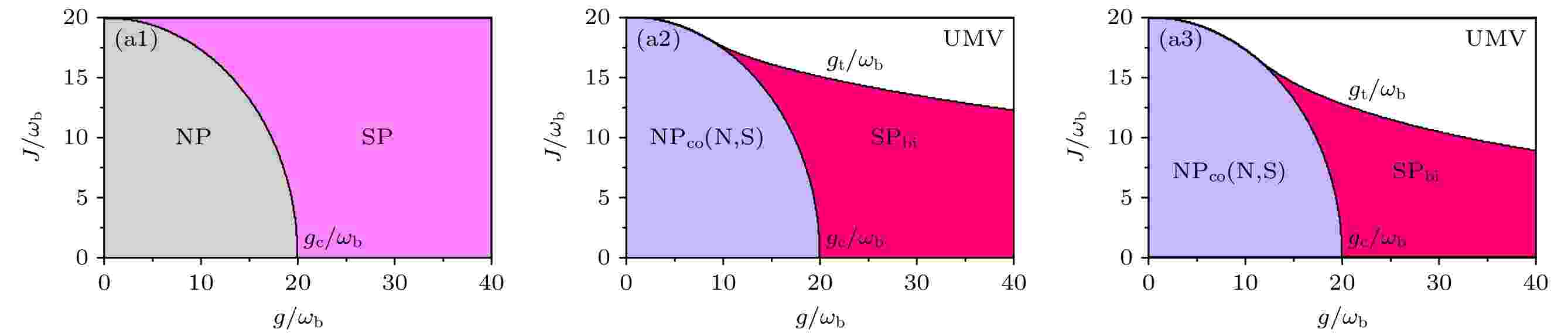
2025, 74 (10): 100202.
doi: 10.7498/aps.74.20250046
Abstract +
In this paper, the quantum phase transitions caused by the interactions between light and atoms, as well as between light and mechanical oscillators, are discussed theoretically in a coupled optomechanical cavity containing two optical field modes (N atoms and mechanical oscillator). By using Holstein-Primak off transformation method, new translational boson operators and four parameters are assumed. The ground state energy functional of the system and a set of equations composed of four parameters are given. The correctness of the assumed translation boson operators is proved by two special cases. In the case of resonance, the characteristics of the obtained solutions are shown by solving the equations, graphical method and Hessian matrix judgment. The stable zero solution is called the normal phase, the unstable zero solution is named the unstable vacuum macroscopic phase, and the stable non-zero solution is referred to as the superradiation phase. The phase can transition from normal phase to superradiation phase, and the point of phase transition can be changed by adjusting the coupling intensity of the two cavity light fields. When the nonlinear photon-phonon interaction caused by radiation pressure is considered, the phase diagram of the system is expanded from the original two phase regions to three phase regions, including the coexistent normal phase and superradiation phase, the bistable superradiation phase, and the unstable vacuum macroscopic phase region, where the bistable superradiation phase is similar to the optical bistable phenomenon. At the same time, there is also a turning point curve, which overlaps with the phase transition point curve, indicating the existence of multiple quantum phase transitions in the system. These predictions can be detected by measuring the average number of photons. The coupled optomechanical cavity that we studied, when considering the coupling of the two-mode optical field and the atomic ensemble but no mechanical oscillator, reflects the interaction between the two-mode optical field and the atom, thus concluding that the transformation point is small and the quantum phase transition is easy to occur. When the coupling between the mechanical oscillator and the two-mode optical field is not considered, the interaction between the single-mode optical field and the atom is reflected, returning to the quantum phase transition of the Dicke model under rotating wave approximation.
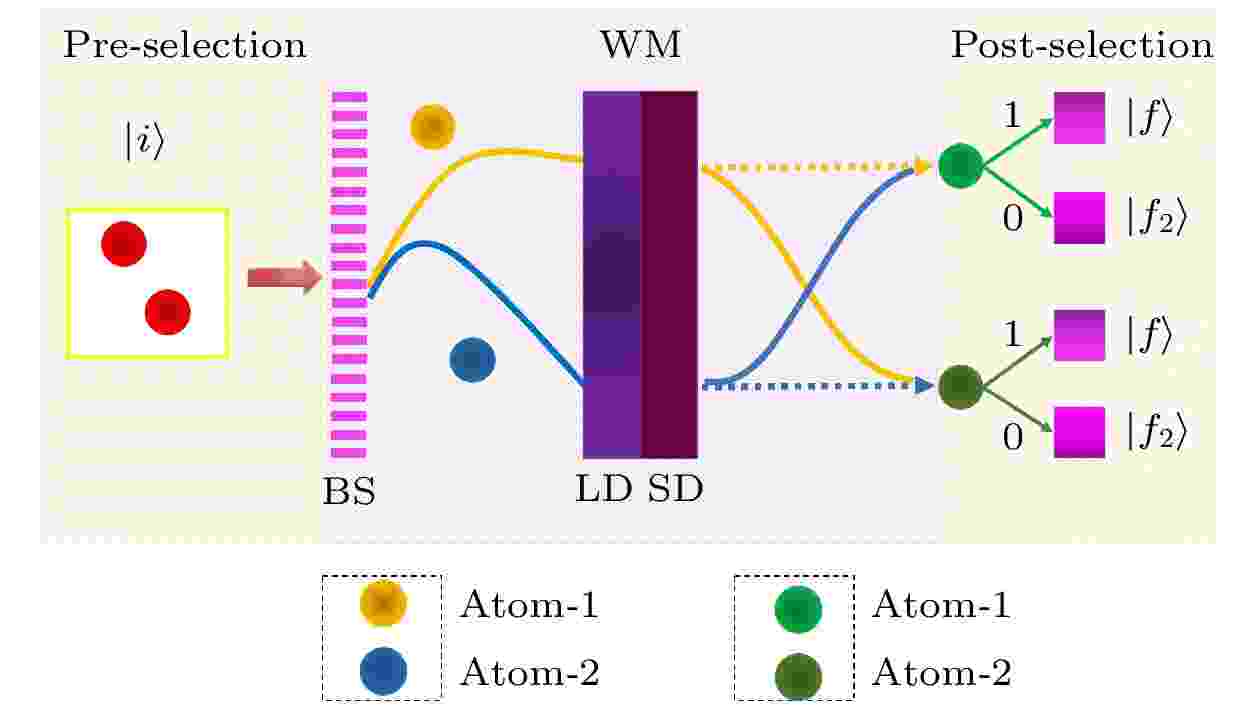
2025, 74 (10): 100301.
doi: 10.7498/aps.74.20241781
Abstract +
The quantum Cheshire cat effect is an important phenomenon in quantum mechanics that reveals the separability of physical properties from their carriers. This effect transcends the classical framework whose attributes must be inherently attached to objects, providing new perspectives for quantum information and precision measurement. According to the quantum Cheshire cat effect, we prepare a pre-selected state of a spin-1/2 atomic system composed of two particles through a pre-selection process. We conduct quantum weak measurements on the spins and positions of these two atoms and extract weak values by using the method of imaginary time evolution (ITE). Subsequently, we perform post-selection on these two atoms and design two distinct post-selected states. Initially, we calculate analytical solutions when both atoms encounter these two different post-selected states separately. Then, during the stage of obtaining weak values via ITE, we first discuss the scenario with only one post-selected state. In this case, our experimental goal is to achieve spin exchange between the two atoms. We use ITE to obtain normalized coincidence rate for the system. By linearly fitting these normalized coincidence rate, we derive numerical solutions for the weak values of the system. The comparison between the analytical solutions and numerical results indicates that they are in close agreement, demonstrating that our method promotes spin exchange between the two atoms. Next, we examine scenarios involving both post-selected states in the post-selection process. After completing weak measurements on particles, delayed-choice allows them to evolve along different paths ultimately leading to distinct post-selected states. One particular post-selected state that results in final measurement outcomes indicates that the spin exchange occurs between both particles with amplification. Conversely, the other post-selected state ensures that even after undergoing weak measurement and delayed-choice, the states of the two particles remain consistent with their pre-measurement conditions. We also compare the analytical and numerical solutions of the experiment involving delayed choice and find that they are very consistent with each other. This consistency indicates that delayed-choice indeed has a significant influence on whether the final exchange occurs. Our research theoretically confirms the feasibility of fermionic systems within bipartite quantum Cheshire cat effects and illustrates how delayed-choice influences quantum Cheshire cat effects in spin-1/2 atomic systems.
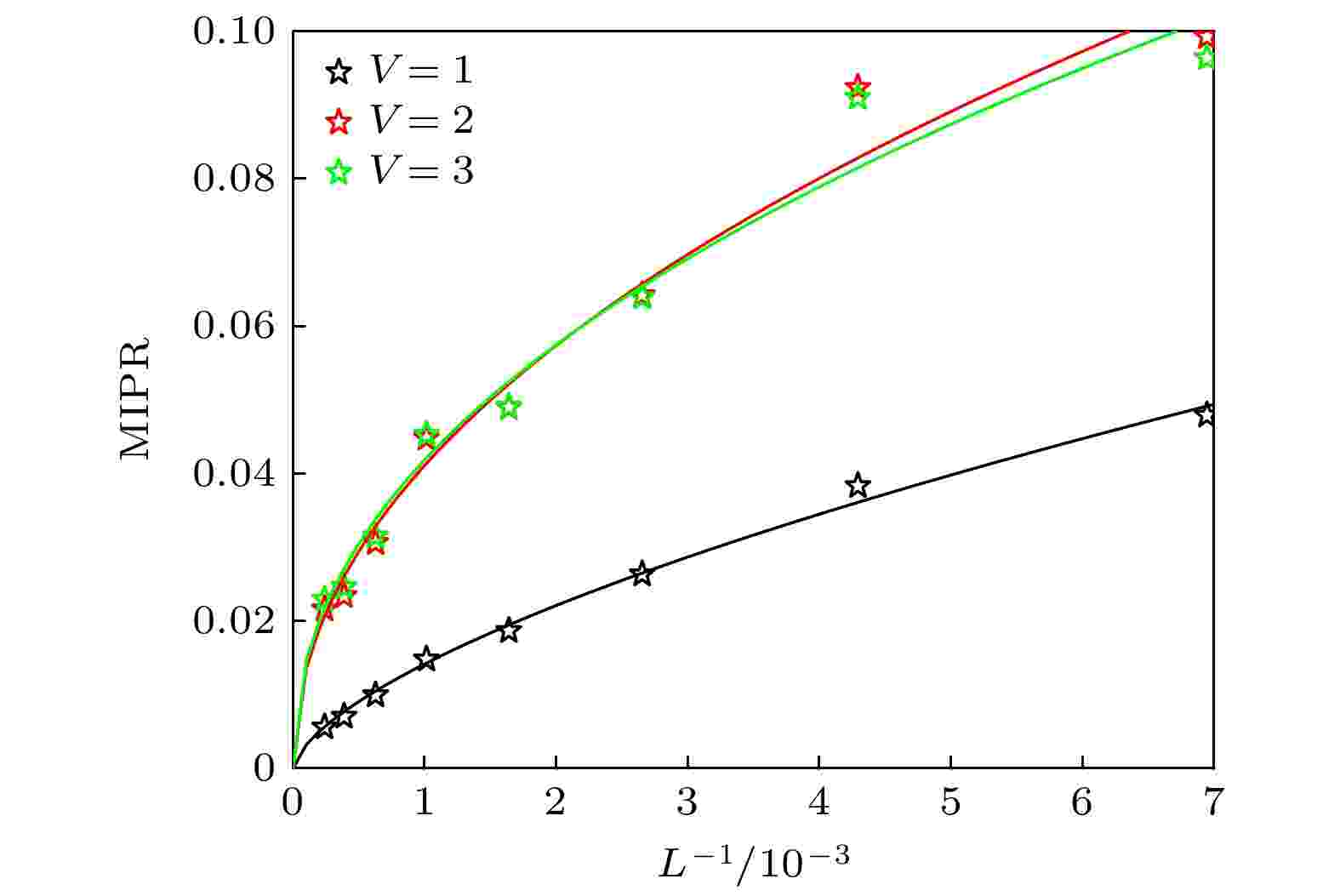
EDITOR'S SUGGESTION
2025, 74 (10): 100303.
doi: 10.7498/aps.74.20250137
Abstract +
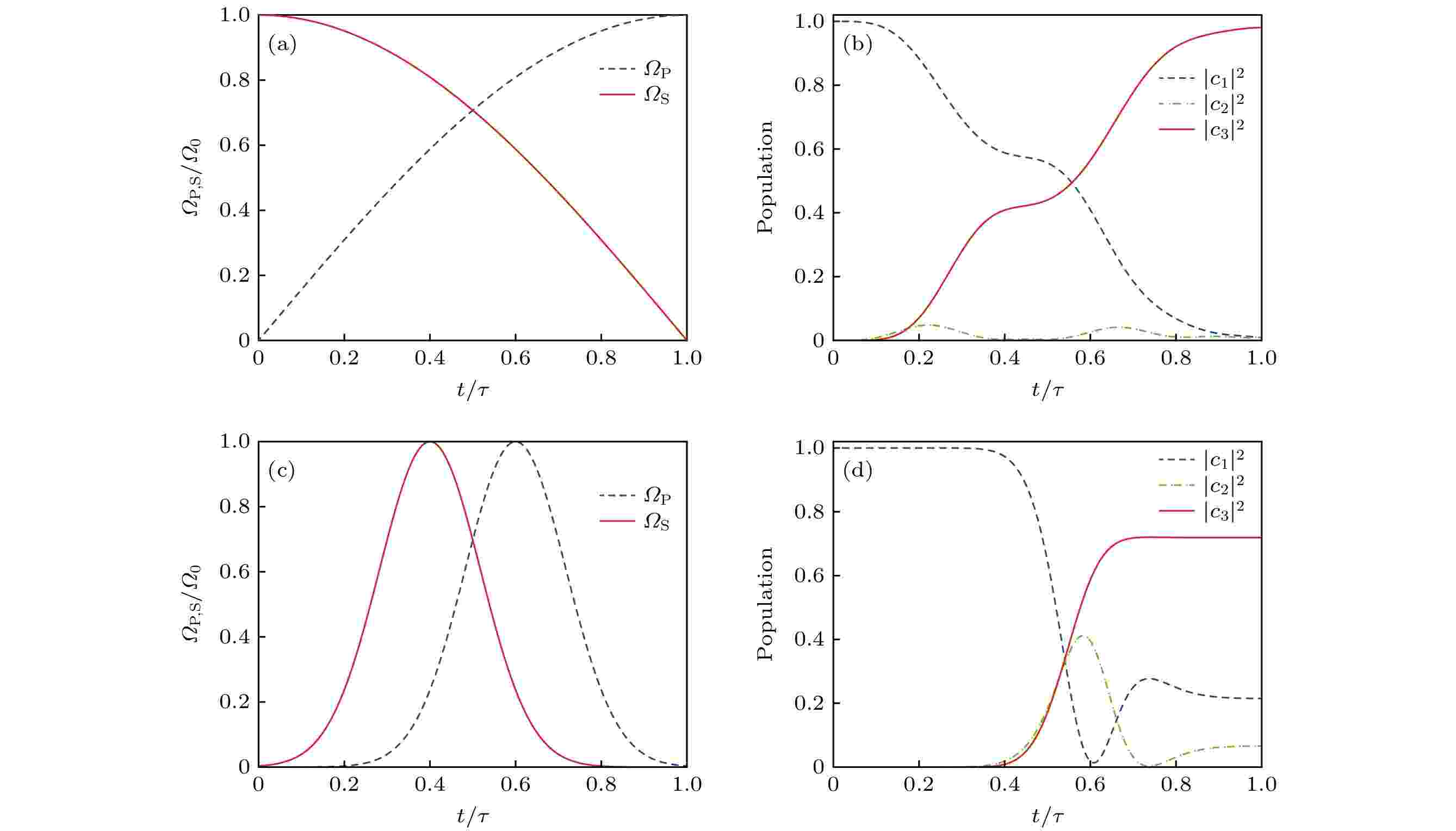
2025, 74 (10): 100304.
doi: 10.7498/aps.74.20250210
Abstract +
The optimal control of population transfer for multi-level systems is investigated from the perspective of quantum geometry. Firstly, the general theoretical framework of optimizing the STIRAP scheme based on the dynamical quantum geometric tensor is given, and then the dynamical quantum geometric tensor and the nonadiabatic transition rate are calculated by taking the detuned $ {{\Lambda }} $-type three-level system and tripod-type four-level system for example. Secondly, the transfer dynamics of the particle population of the system are investigated in detail. For a three-level system, the optimal STIRAP scheme has an efficiency of over 98% in transferring the population to the state $ \left|3\right.\rangle $, while the transfer efficiency of traditional STIRAP is about 72%. The superposition states with arbitrary proportions can be efficiently prepared for a four-level system due to the decoupling of the degenerate dark states. Finally, the influences of system parameters, such as the operating time of the Rabi pulses, the amplitude fluctuation and the single-photon detuning, on the transfer process are discussed. Especially, the phenomena of the adiabatic resonance transfer are revealed. Choosing the pulse parameters in the resonance window can reduce the infidelity of the population transfer to below 10–3. It is found that the optimal STIRAP scheme by the dynamical quantum geometric tensor provides faster and more efficient transfer than the traditional STIRAP scheme.
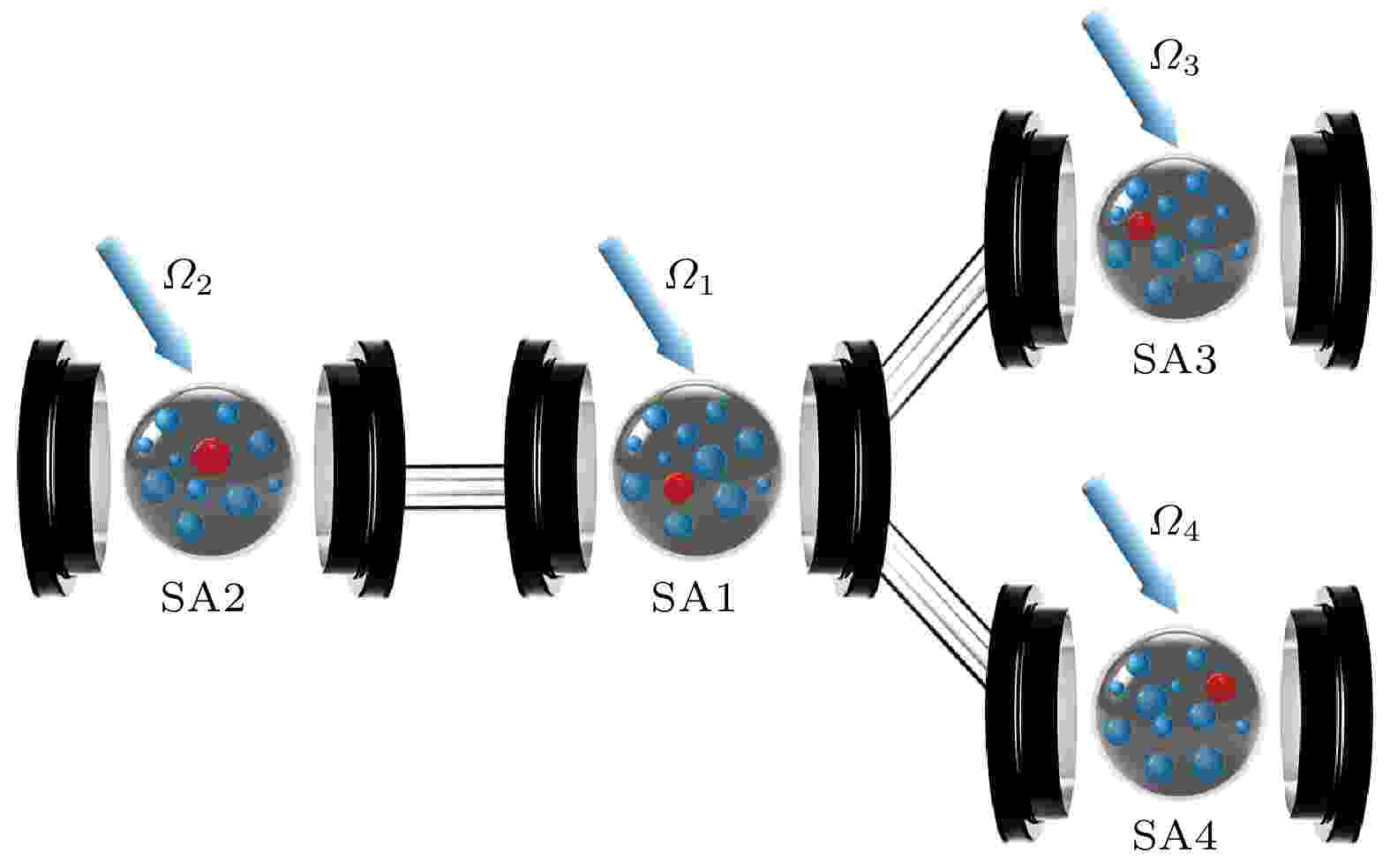
EDITOR'S SUGGESTION
2025, 74 (10): 100305.
doi: 10.7498/aps.74.20241694
Abstract +
The W state, as a robust multipartite entangled state, plays an important role in quantum information processing, quantum network construction and quantum computing. In this paper, a three-level ladder-type Rydberg atomic system is placed into a Rydberg blocking sphere to form a superatom. Each superatom has many collective states including just one Rydberg excitation constrained by the Rydberg blockade effect. In the weak cavity field limit, at most one atom can be pumped into excited state, then we can describe the superatom by using a three-level ladder-type system. Afterwards we encode quantum information about the effective energy levels of Rydberg superatoms and propose a fast scheme for preparing the Rydberg superatom W state based on the superadiabatic iterative technique and quantum Zeno dynamics.This scheme can be achieved in only one step by controlling the laser pulses. In this scheme, the superatoms are trapped in spatially separated cavities connected by optical fibers, thereby greatly improving the feasibility of experimental manipulation. A remarkable feature is that it does not need to accurately control experimental parameters and interaction time. Meanwhile, the form of counterdiabatic Hamiltonian is the same as that of the effective Hamiltonian. Through numerical simulations, the fidelity of this scheme can reach 99.94%. Even considering decoherence effects, including atomic spontaneous emission and photon leakage, the fidelity can still exceed 97.5%, thereby further demonstrating the strong robustness of the solution. In addition, the Rabi frequency can be characterized as a linear superposition of Gaussian functions, and this representation significantly alleviates the complexity encountered in practical experiments. Futhermore, we also analyze the influence of parameter fluctuations on the fidelity, and the results show that this scheme is robust against parameter fluctuations. Finally, the present scheme is extended to the case of N Rydberg superatoms, which shows the scalability of our scheme.
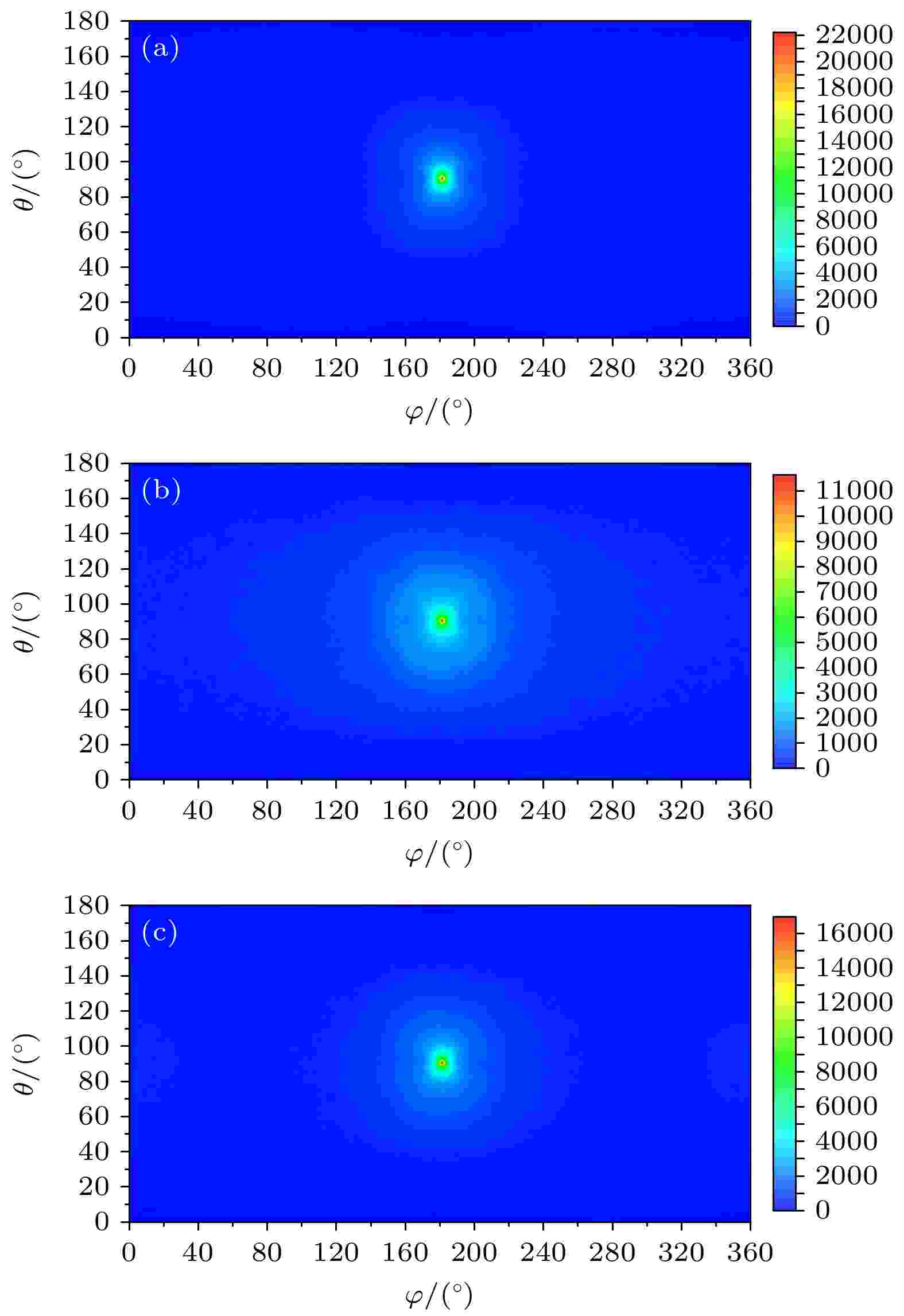
2025, 74 (10): 100701.
doi: 10.7498/aps.74.20241723
Abstract +
The Compton camera for γ-ray imaging has the advantages of light weight, high detection efficiency, and wide imaging energy range. However, it is difficult for the detection system to distinguish between the Compton scattering event and scattering photon absorption event, which results in erroneous image reconstruction. In this work, a simulation model of Compton camera based on a three-dimensional position-sensitive CdZnTe detector is constructed using GEANT4 program. The detection of characteristic γ-ray from a far-field 137Cs point-like source is simulated. The location of the interaction and energy deposition in the detector are recorded by means of event-by-event. The Compton scattering angles of effective Compton scattering events and imaging of the radioactive source are reconstructed using a simple back-projection algorithm which is an image reconstruction algorithm suitable for real-time imaging scenes. The influences of event sequence reconstruction on the imaging resolution and its improvement are investigated. The results show that the influence of incorrect sequence events on imaging resolution is mainly in the area within 30° deviation from the source position, resulting in a decrease in the density of the image points distributed at the source position. Incorrectly reconstructed image points are generated near the source position and form a ring at 26°. The percentage of correctly sequenced events increases to 82% by using Compton edge test and simple comparison method based on the deposited energy for sequencing events. The density of image points distributed at the source location is improved by 47%, and the incorrect reconstruction of the image point distribution near the source location is greatly suppressed, resulting in an improved imaging resolution. The research results provide support for designing Compton camera and optimizing image reconstruction.
COVER ARTICLE
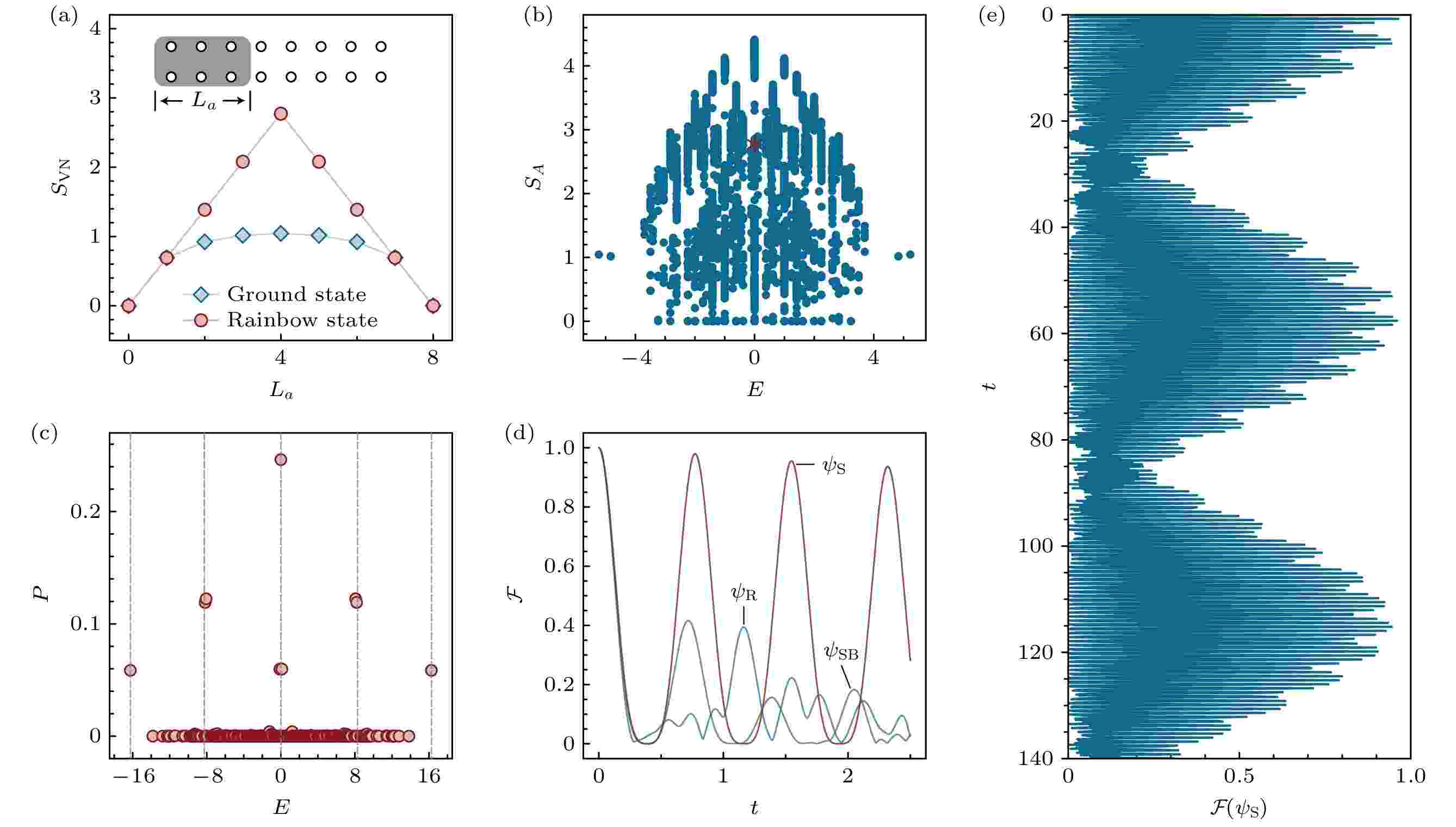
COVER ARTICLE
2025, 74 (10): 100302.
doi: 10.7498/aps.74.20250061
Abstract +
The eigenstate thermalization hypothesis describes the nonequilibrium dynamics of an isolated quantum many-body system, during which a pure state becomes locally indistinguishable from a thermal ensemble. The discovery of quantum many-body scars (QMBS) shows a weak violation of ergodicity, characterized by coherent oscillations of local observables after a quantum quench. These states consist of the tower of regular eigenstates which are equally spaced in the energy spectrum. Although subextensive entanglement scaling is a primary feature widely used to detect QMBS numerically as entropy outliers, rainbow scars exhibit volume-law scaling, which may challenge this property. In this work, we construct the rainbow scar state in the fracton model on a two-leg ladder. The fracton model is composed of four-body ring-exchange interactions, exhibiting global time-reversal symmetry $ \hat{{{\cal{T}}}}={{\cal{K}}} {\mathrm{i}} \hat{\sigma}^y $ and subsystem $ {\hat{U}(1)}=\displaystyle \prod\nolimits_{j \in \{\text {row/col}\}} {\mathrm{exp}}\Big({{\mathrm{i}} \dfrac{\theta}{2} \hat{\sigma}_j^z}\Big)$ symmetry. The subsystem symmetry constrains particle mobility, hindering the establishment of thermal equilibrium and leading to a series of anomalous dynamical processes. We construct the rainbow scar state with distributed four-body GHZ states whose entanglement entropy follows the volume law. By calculating the eigenstates of the fracton model with exact diagonalization, the rainbow scar state consists of a series of degenerate high-energy excited states that are not significant outliers among other eigenstates in the spectrum. By introducing the on-site interaction to break the time-reversal symmetry, the degeneracy of rainbow scar states is lifted into an equally spaced tower of states, ensuring the revival of the initial state. However, when subsystem $\hat U(1) $ symmetry is broken, the scar state is quickly thermalized, indicating that the weak thermalization may be protected by subsystem $\hat U(1) $ symmetry. Additionally, we propose a scheme for preparing the rainbow scar state by modulating the strength of the four-body interaction and $ \hat{\sigma}^z$ operations, analyzing the influence of noise on the strength of the four-body interaction. This work provides new insights into the weak thermalization processes in fracton model and aids in understanding the nature of ETH-violation in various nonequilibrium systems.
NUCLEAR PHYSICS
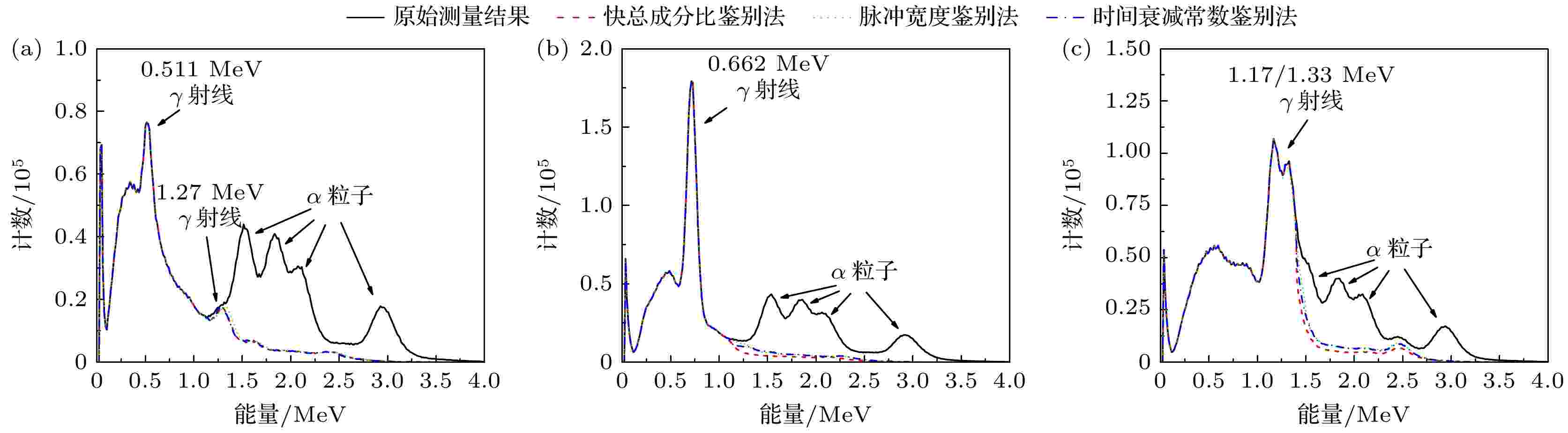
EDITOR'S SUGGESTION
2025, 74 (10): 102801.
doi: 10.7498/aps.74.20250017
Abstract +
ATOMIC AND MOLECULAR PHYSICS
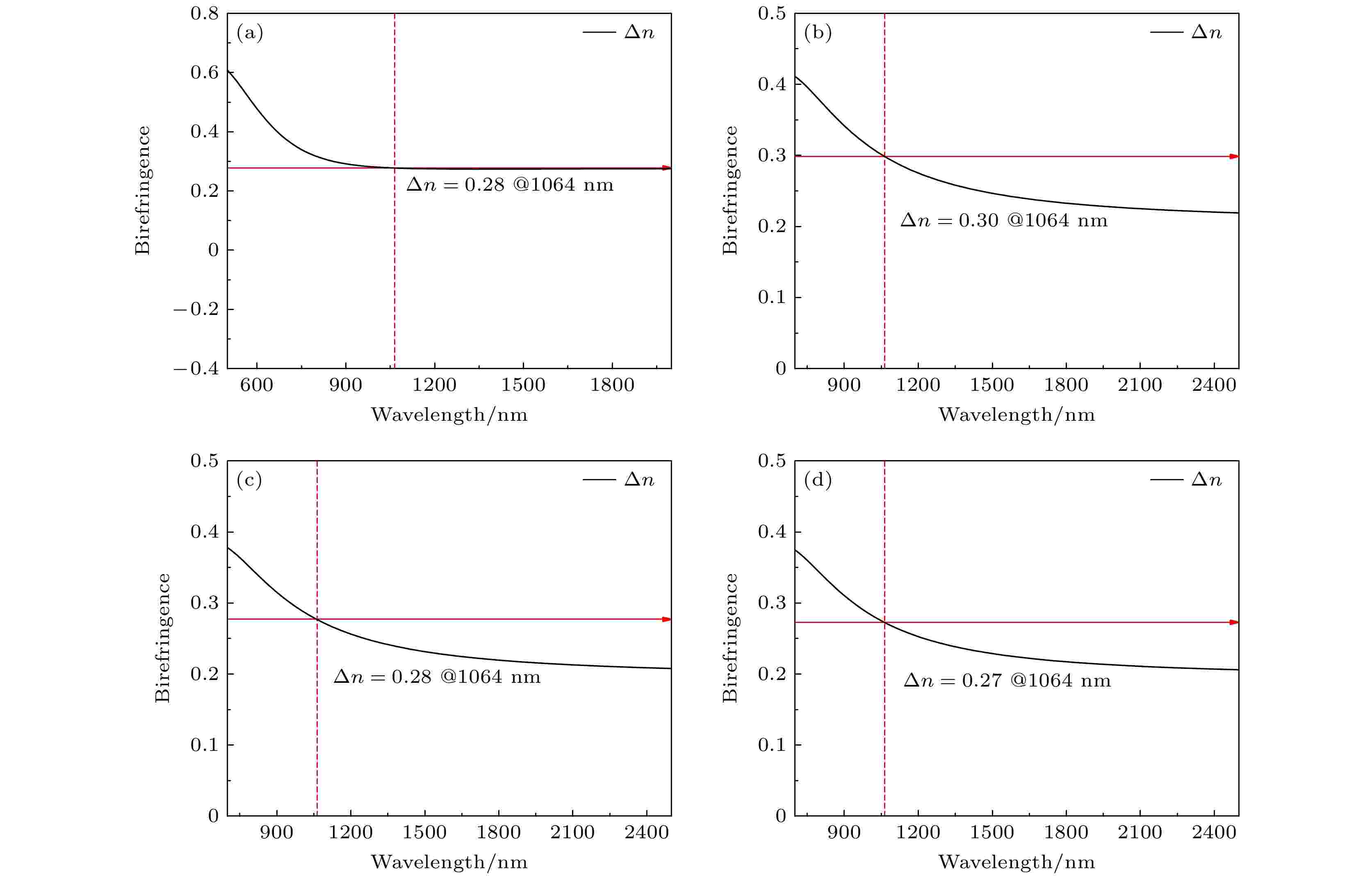
2025, 74 (10): 103101.
doi: 10.7498/aps.74.20241631
Abstract +
Birefringence, as a fundamental parameter of optical crystals, plays a vital role in numerous optical applications such as phase modulation, light splitting, and polarization, thereby making them key materials in laser science and technology. The significant birefringence of vanadate polyhedra provides a new approach for developing birefringent materials. In this study, first-principles calculations are used to investigate the band structures, density of states (DOS), electron localization functions (ELFs), and birefringence behaviors of four alkali metal vanadate crystals AV3O8 (A = Li, Na, K, Rb). The computational results show that all AV3O8 crystals have indirect band gaps, whose values are 1.695, 1.898, 1.965, and 1.984 eV for LiV3O8, NaV3O8, KV3O8, and RbV3O8, respectively. The DOS analysis reveals that near the Fermi level, the conduction band minima (CBM) in these vanadates are predominantly occupied by the outermost orbitals of V atoms, while the valence band maxima (VBM) are primarily contributed by O-2p orbitals. The O-2p orbitals also exhibit strong localization near the Fermi level. Combined with highest occupied molecular orbital-lowest unoccupied molecular orbital (HOMO-LUMO) analysis and population analysis, the bonding interactions in all four crystals mainly arise from the hybridization between V-3p and O-2p orbitals, indicating strong covalent bonding in V—O bonds. Through the analysis of structure-property relationships, the large birefringence is primarily attributed to the pronounced structural anisotropy, high anisotropy index of responsive electron distribution, unique arrangement of anionic groups, and d-p orbital hybridization between V-3d and O-2p orbitals. The calculated birefringence values at a wavelength of 1064 nm for LiV3O8, NaV3O8, KV3O8, and RbV3O8 are 0.28, 0.30, 0.28, and 0.27, respectively.
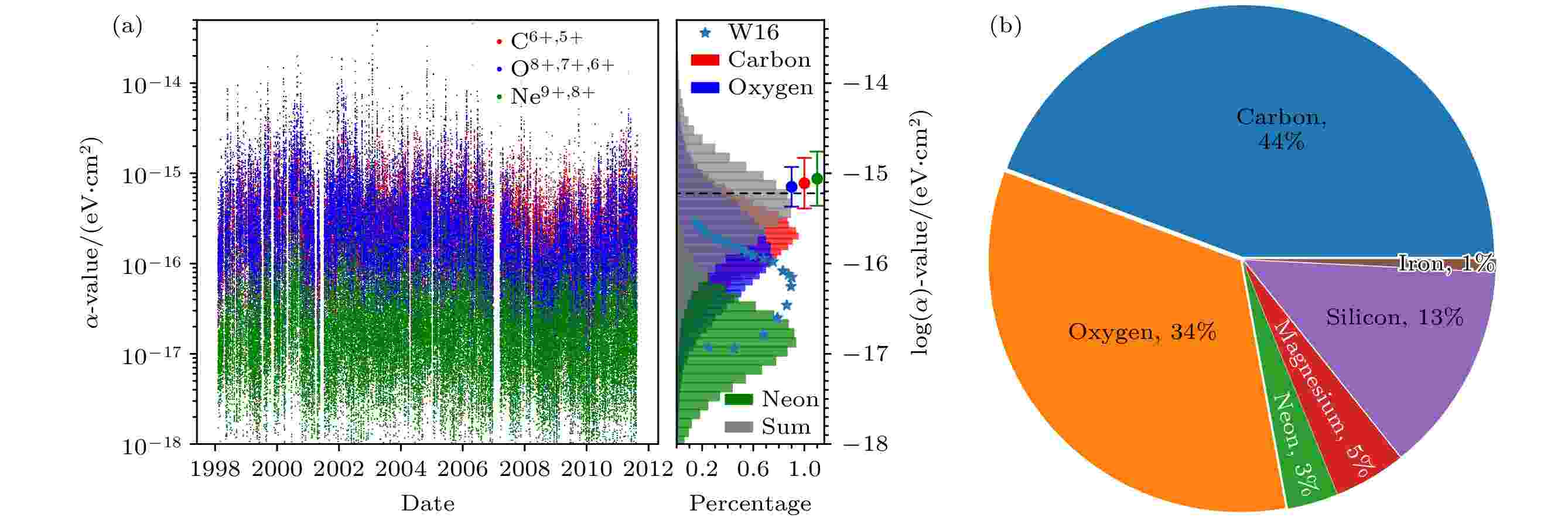
2025, 74 (10): 103201.
doi: 10.7498/aps.74.20241603
Abstract +
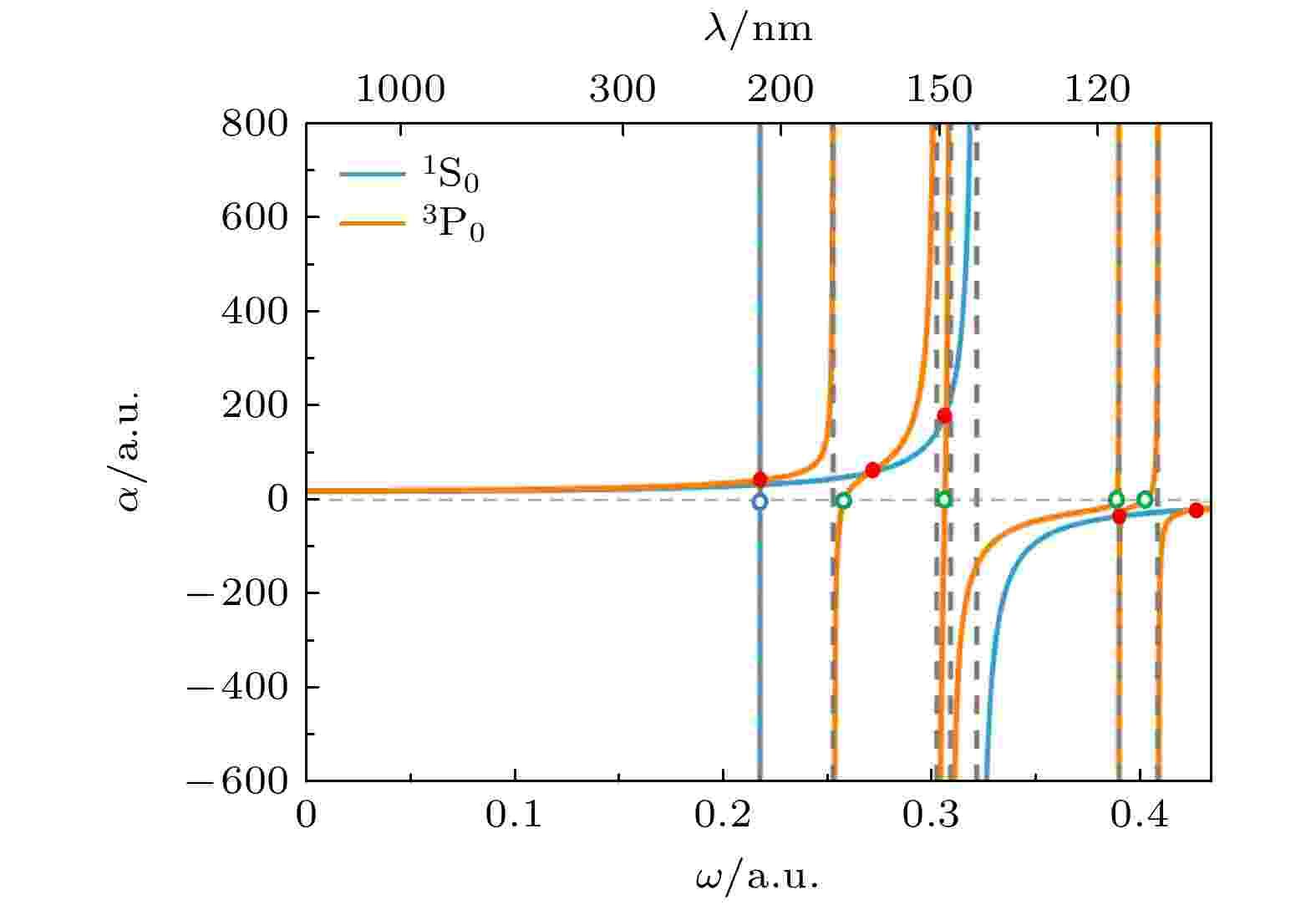
2025, 74 (10): 103202.
doi: 10.7498/aps.74.20250125
Abstract +
The transition of Ga+ ions from 4s2 1S0 to 4s4p 3P0 has advantages such as a high quality factor and a small motional frequency shift, making it suitable as a reference for precision measurement experiments like optical clocks. Calculating the dynamic polarizability of 4s2 1S0—4s4p 3P0 transition for Ga+ ion is of great significance for exploring the potential applications of the Ga+ ion in the field of quantum precision measurement and for testing atomic and molecular structure theories. In this paper, the dynamic polarizability of the Ga+ ion 4s2 1S0—4s4p 3P0 transition is theoretically calculated using the relativistic configuration interaction plus many-body perturbation (RCI+MBPT) method. The “tune-out” wavelengths for the 4s2 1S0 state and the 4s4p 3P0 state, as well as the “magic” wavelength of the 4s2 1S0—4s4p 3P0 transition, are also computed. It is observed that the resonant lines situated near a certain “turn-out” and “magic” wavelength can make dominant contributions to the polarizability, while the remaining resonant lines generally contribute the least. These “tune-out” and “magic” wavelengths provide theoretical guidance for precise measurements, which is important for studying the atomic structure of Ga+ ions. The accurate determination of the difference in static polarizability between the 4s2 1S0 and 4s4p 3P0 states is of significant importance. Additionally, based on the “polarizability scaling” method, this work also discusses how the theoretical calculation errors in static polarizability measurements vary with wavelength, which provides theoretical guidance for further determining the static polarizability of the 4s2 1S0 and 4s4p 3P0 states with high precision. This is crucial for minimizing the uncertainty of the blackbody radiation (BBR) frequency shift in Ga+ optical clock and suppressing the systematic uncertainty.
ELECTROMAGNETISM, OPTICS, ACOUSTICS, HEAT TRANSFER, CLASSICAL MECHANICS, AND FLUID DYNAMICS
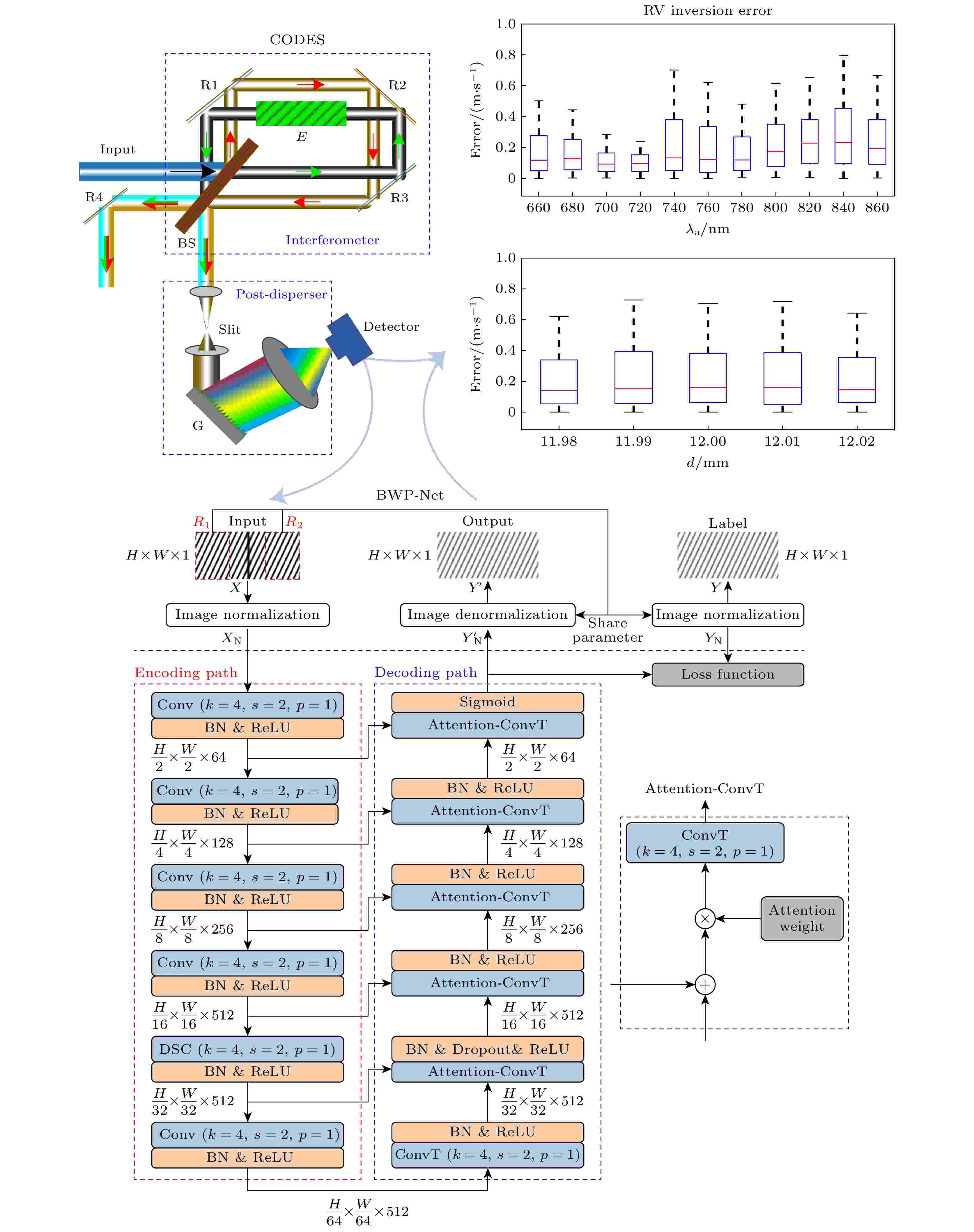
2025, 74 (10): 104201.
doi: 10.7498/aps.74.20250090
Abstract +
Coherent-dispersion spectrometer (CODES) is an exoplanet detection instrument based on the radial velocity (RV) method. It detects changes in RV by measuring the Doppler phase shift of the interference spectrum of stellar absorption line. However, the background white light in the stellar absorption spectrum disturbs the phase analysis of CODES, which leads to phase error and seriously affects the accuracy of RV inversion. The larger the cosine amplitude of the background white light, the greater the error is. In order to effectively remove background white light and correct Doppler phase shift, a background white light prediction network (BWP-Net) is proposed based on the U-Net architecture by utilizing the principle and data characteristics of CODES in this study. To accelerate the convergence of the BWP-Net model, the interference spectrum of absorption line from CODES and the ideal interference spectrum of background white light are used as inputs and labels for the model after image normalization, while the model output becomes the predicted interference spectrum of background white light after inverse normalization. The BWP-Net consists of symmetric 6-layer encoding path and decoding path. First, in the encoding path, different levels of features are extracted step by step from the interference spectrum of stellar absorption line through combination of multi-channel convolution and depthwise separable convolution, extracting features effectively while reducing computational costs reasonably. In each convolution layer, spatial downsampling is performed through convolution with a stride of 2 and the number of feature channels is increased until the fourth layer, thus various features, from simple to abstract, local to global, are extracted for the preparation of image reconstruction in the decoding path. Second, in the decoding path, the image details are gradually reconstructed from the features extracted through several layers of attention transposed-convolution. In each layer of attention transposed-convolution, spatial upsampling is performed based on the fusion of shallow features and deep features through matrix addition and the number of feature channels are reduced, at the same time attention of different levels is paid to the features through a learnable weight matrix, so as to suppress the absorption line information gradually during image reconstruction. At the last layer of the decoding path, the sigmoid activation function is used to control the model output in the 0-1 interval, making it easier to denormalize. Finally, a region weighted loss function that combines mean-square error and multi-scale structural similarity is used for training so as to consider pixel level differences and structural similarity between the model output and the labels, while enhancing the suppression of absorption lines in the central region of the interference spectrum through region weighting. And the output of BWP-Net is the prediction of the interference spectrum of background white light, which is subtracted from the interference spectrum of stellar absorption lines for phase analysis. The experimental results show that under different absorption lines, different fixed optical path differences, and different RVs, after removing background white light from the output of BWP-Net, the RV inversion error is less than 1 m/s, mainly concentrated in the region of 0–0.4 m/s, with an average error of 0.2353 m/s and a root mean square error of 0.3769 m/s. And the distribution of RV inversion error is relatively uniform under different parameter conditions, the median error is less than 0.25 m/s at different absorption line wavelengths, and less than 0.2 m/s at different fixed optical path differences. Thes indicate that BWP-Net not only predicts background white light accurately, but also has good stability and robustness, providing strong support for high-precision and stable RV inversion for CODES.
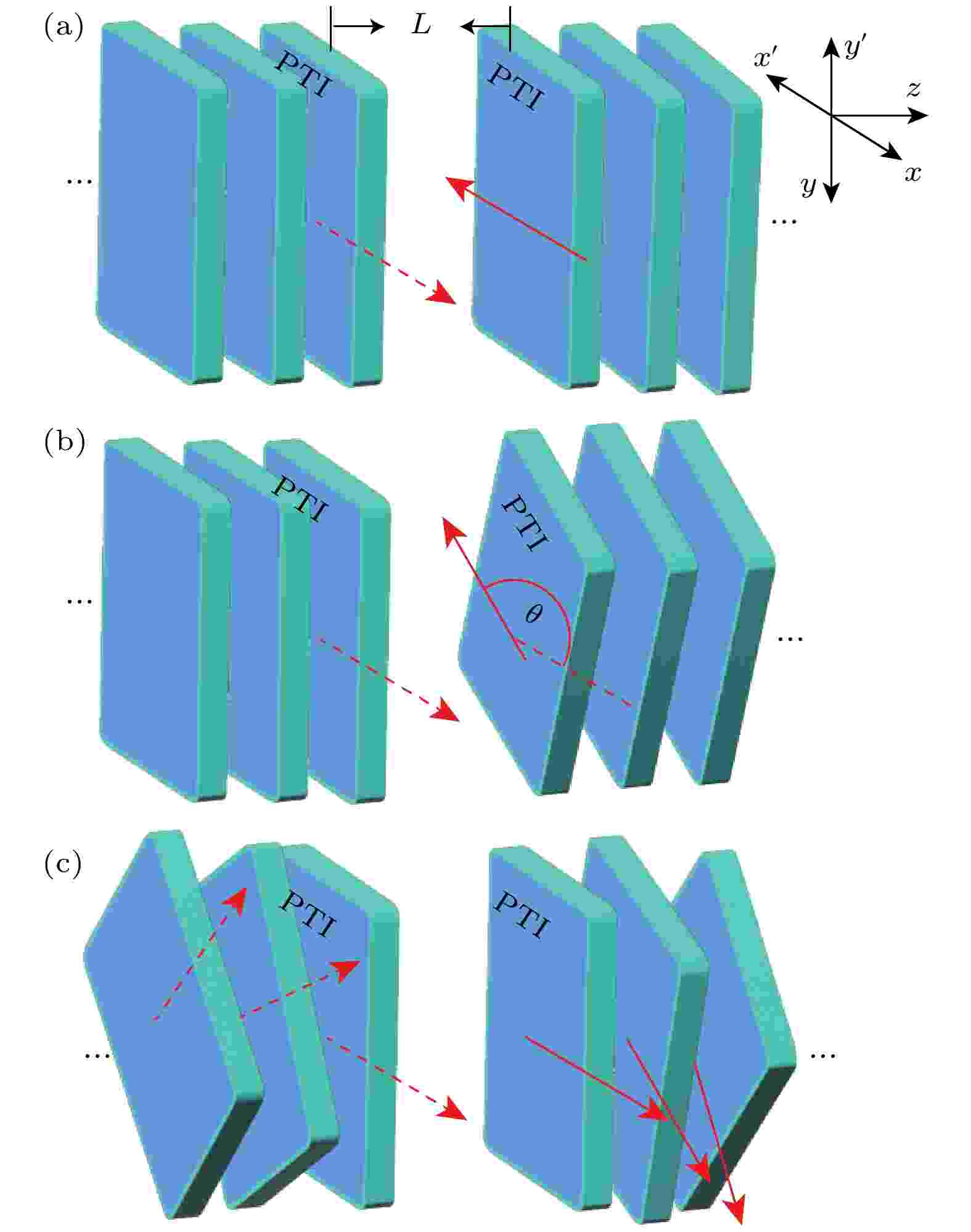
EDITOR'S SUGGESTION
2025, 74 (10): 104202.
doi: 10.7498/aps.74.20250088
Abstract +
The Casimir effect has received extensive attention theoretically and experimentally in recent years. It arises from the macroscopic manifestation of quantum vacuum fluctuations, and this Casimir interaction force can be an effective means of driving and controlling components in micro-electro-mechanical system (MEMS) and nano-electromechanical system (NEMS). Due to the new possibilities provided by photonic topological insulator for designing and using photonic devices, in this work, the Casimir force between the multilayer structures of non-reciprocal photonic topological insulators with broken time-reversal symmetry is investigated, and the influences of the dielectric tensor of the photonic topological insulator, the spatial structural parameters of the multilayer system, and the rotational degree of freedom on the Casimir force are examined. It is found that there exists Casimir repulsive force in such a multilayer system, and the Casimir stable equilibrium and restoring force can be further realized and controlled. Continuous variation between anti-mirror-symmetric configuration and mirror-symmetric configuration is examined. Both the Casimir attraction and repulsion can be generally enhanced through structural optimization by increasing layer number and individual layer thickness. Furthermore, we focus on the detailed analysis of how the optical axis angle difference within the photonic topological insulator layers can be used to adjust the Casimir force. The overall relative rotation of the multilayer system may adjust the magnitude and the direction of the Casimir force, and some inflection points can be found from the influence curve of the optical axis angle difference between internal layers of the multilayer on the Casimir force, allowing the rotational degrees of freedom in the multilayer system to be used for fine-adjusting the Casimir interaction. This work introduces the enhanced degrees of freedom for probing and manipulating the interaction between small objects in micro/nano systems, thereby suppressing adverse Casimir forces and effectively using them.
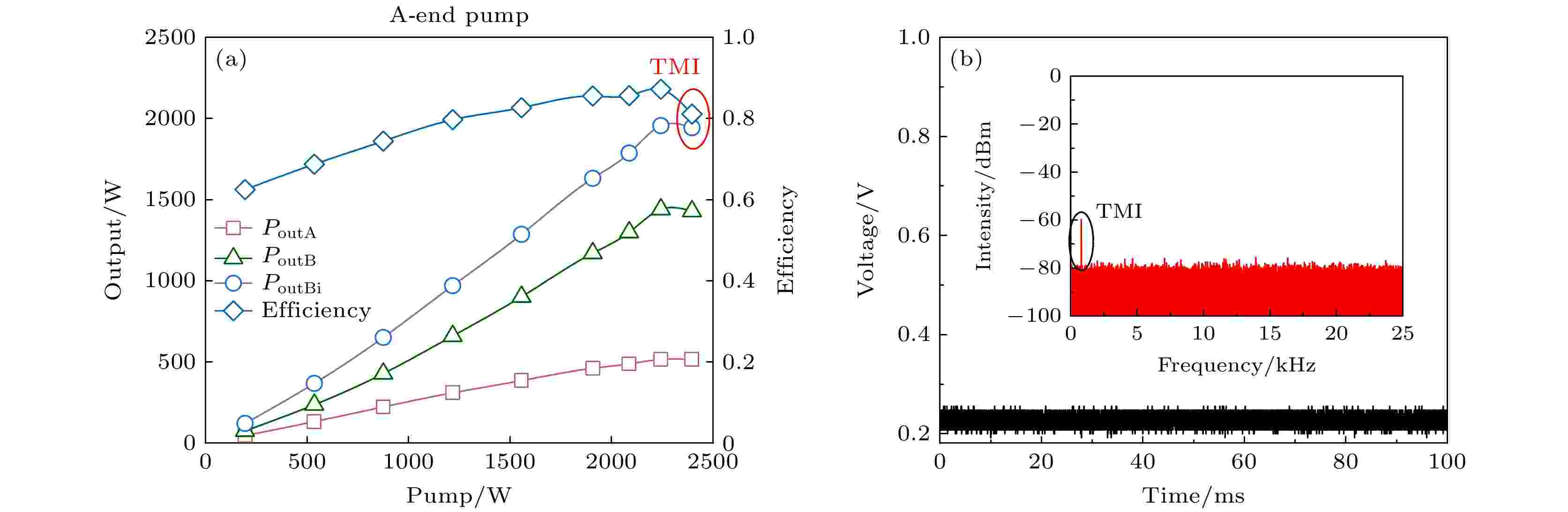
2025, 74 (10): 104203.
doi: 10.7498/aps.74.20250072
Abstract +
High-power fiber laser oscillators have been widely used in industrial processing, material processing, biomedical and other fields due to their compact structure, simple logic and strong power scalability. With the increasing demand for laser performance in industrial applications, bidirectional output fiber laser based on a single resonator structure has a broad application prospect. In this work, we first establish a theoretical model for a 1050-nm bidirectional output fiber laser oscillator based on the steady-state rate equation, and simulate the relationship between the length of the gain fiber and output power, efficiency, and the intensity of stimulated Raman scattering (SRS). A high-power bidirectional output fiber laser with a central wavelength of 1050 nm is built using an ytterbium-doped fiber with a core/cladding diameter of 20/400 μm. The output characteristics of the 1050-nm bidirectional output fiber laser oscillator under different pump methods (unidirectional pump, bidirectional pump) are experimentally studied in detail. With a total pump power of 5262 W, A-end output power reaches 1419 W and B-end output power reaches 3051 W. Therefore, a total output power of 4470 W with an optical-to-optical conversion efficiency of 84.9% is achieved. The corresponding beam qualities (M2 factor) of both ends are 1.27 and 1.31 when the output powers reach 1458 W and 2733 W, respectively. By further optimizing the length of the gain fiber, the amplified spontaneous emission (ASE) and SRS are effectively suppressed. With a total pump power of 5262 W, the Raman suppression ratios at A-end and B-end are enhanced by ~6.6 dB and ~8.1 dB, respectively. It is expected that higher output power can be achieved by increasing the pump power and optimizing the laser structure in the future.
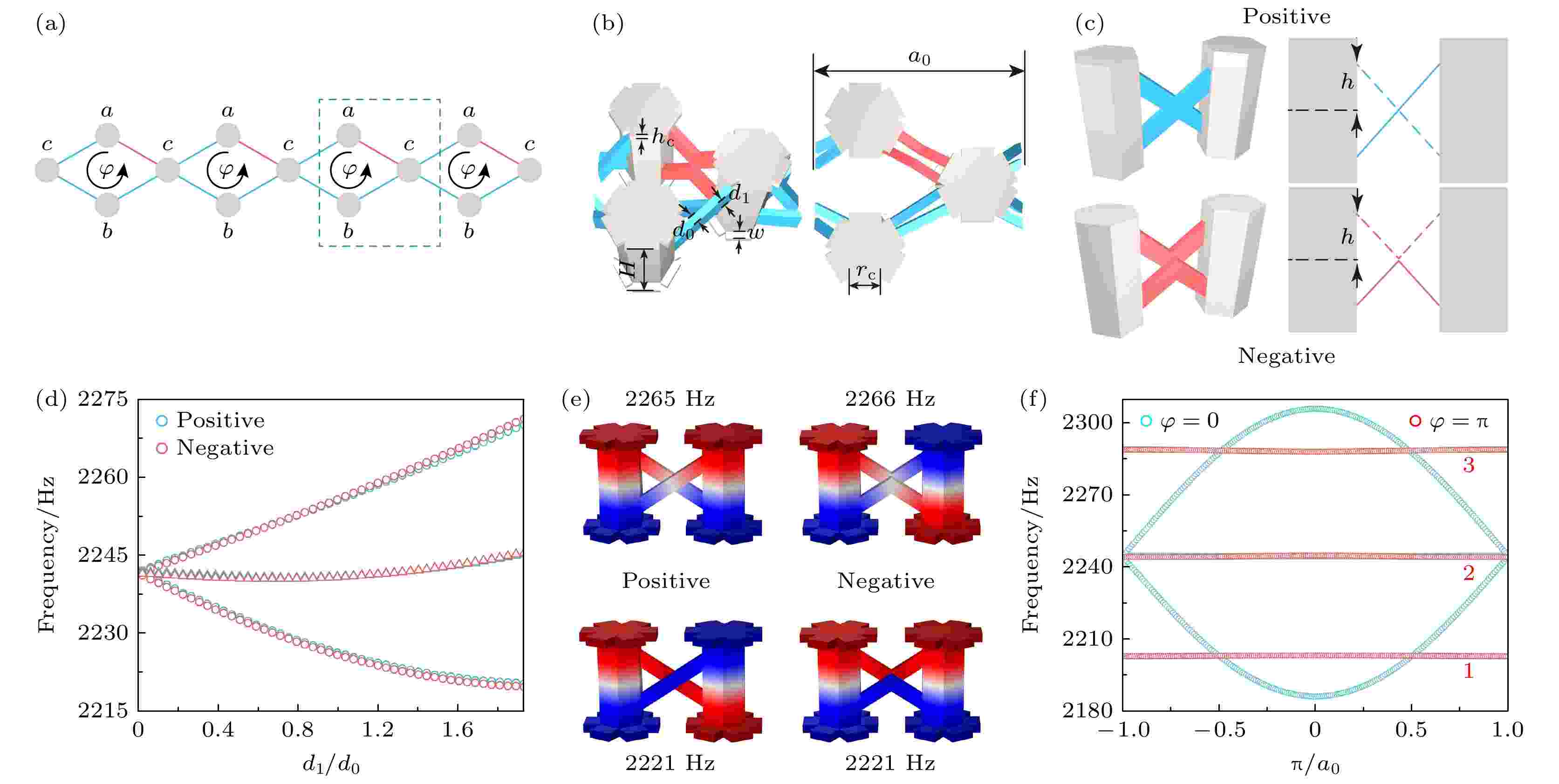
2025, 74 (10): 104301.
doi: 10.7498/aps.74.20241717
Abstract +
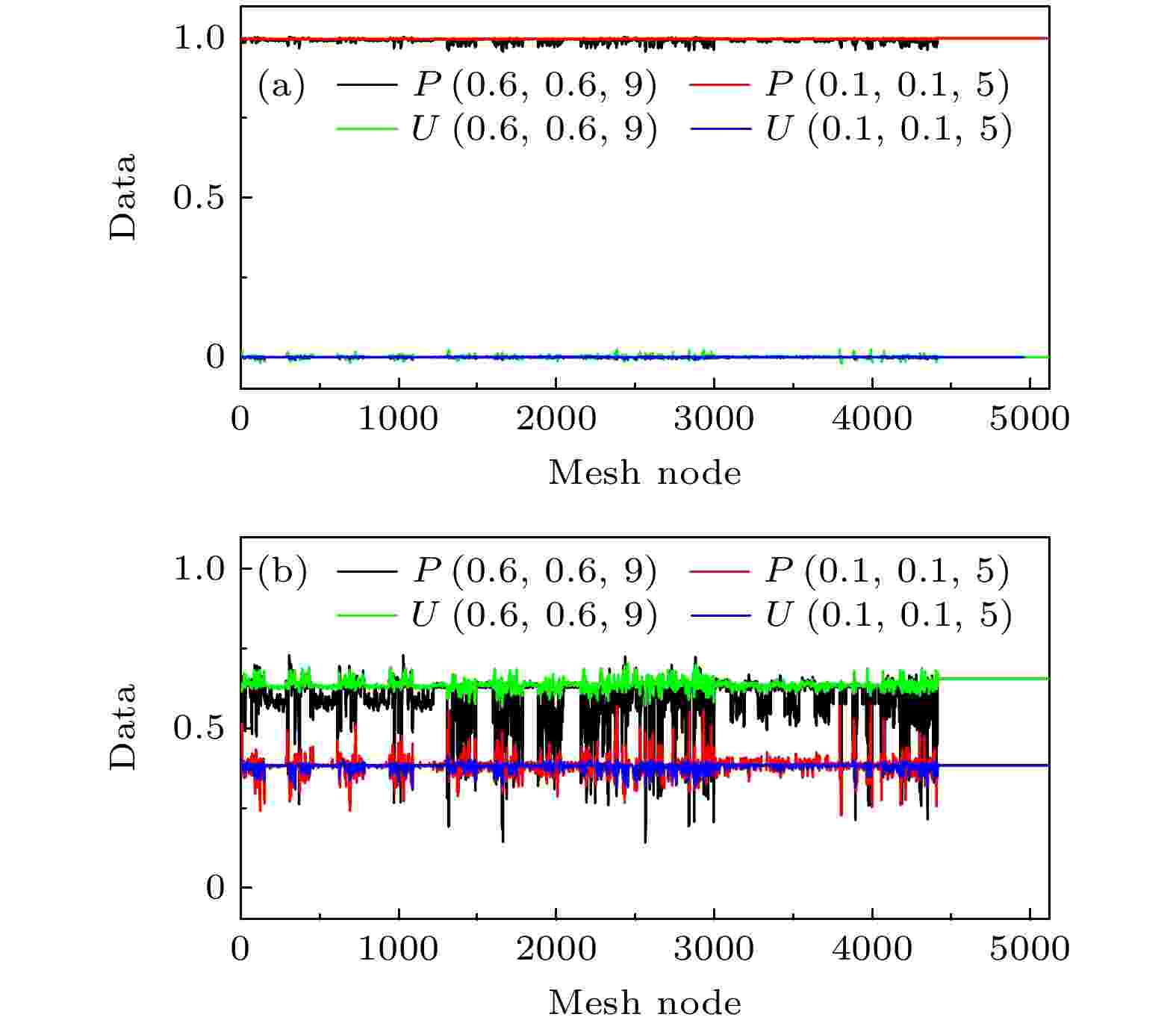
EDITOR'S SUGGESTION
2025, 74 (10): 104701.
doi: 10.7498/aps.74.20241499
Abstract +
The manta ray is a large marine species, which has the ability of gliding efficiently and flapping rapidly. It can autonomously switch between various motion modes, such as gliding, flapping, and group swimming, based on ocean currents and seabed conditions. To address the computational resource and time constraints of traditional numerical simulation methods in modeling the manta ray’s three-dimensional (3D) large-deformation flow field, this study proposes a novel generative artificial intelligence approach based on a denoising probabilistic diffusion model (surf-DDPM). This method predicts the surface flow field of the manta ray by inputting a set of motion parameter variables. Initially, we establish a numerical simulation method for the manta ray’s flapping mode by using the immersed boundary method and the spherical function gas kinetic scheme (IB-SGKS), generating an unsteady flow dataset comprising 180 sets under frequency conditions of 0.3–0.9 Hz and amplitude conditions of 0.1–0.6 body lengths. Data augmentation is then performed. Subsequently, a Markov chain for the noise diffusion process and a neural network model for the denoising generation process are constructed. A pretrained neural network embeds the motion parameters and diffusion time step labels into the flow field data, which are then fed into a U-Net for model training. Notably, a transformer network is incorporated into the U-Net architecture to enable the handling of long-sequence data. Finally, we examine the influence of neural network hyperparameters on model performance and visualize the predicted pressure and velocity fields for multi-flapping postures that were not included in the training set, followed by a quantitative analysis of prediction accuracy, uncertainty, and efficiency. The results demonstrate that the proposed model achieves fast and accurate predictions of the manta ray’s surface flow field, characterized by extensive high-dimensional upsampling. The minimum PSNR value and SSIM value of the predictions are 35.931 dB and 0.9524, respectively, with all data falling within the 95% prediction interval. Compared with CFD simulations, the single-condition simulations by using AI model show that the prediction efficiency is enhanced by 99.97%.
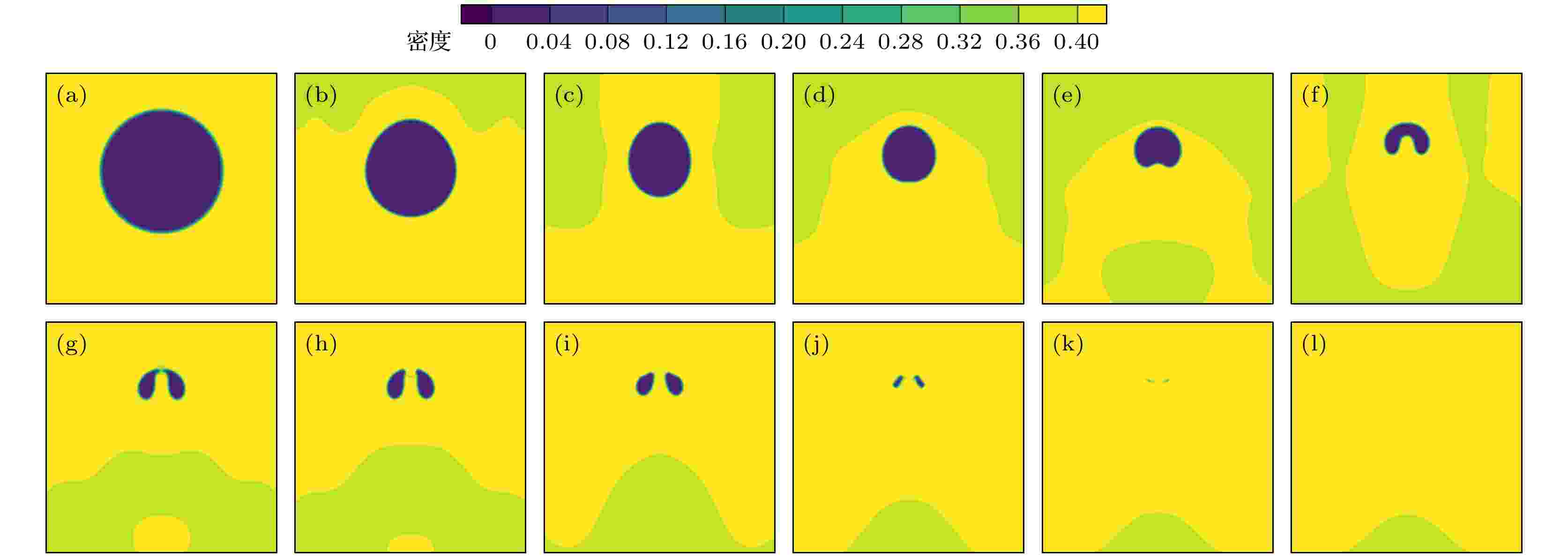
2025, 74 (10): 104702.
doi: 10.7498/aps.74.20241114
Abstract +
To reveal the load mechanism of wall damage induced by bubble collapse, the near-wall cavitation bubble collapse evolution is numerically simulated using an improved multi-relaxation-time lattice Boltzmann method (MRT-LBM), and the dynamic behavior of near-wall cavitation bubble is systematically analyzed. First, the improved multi-relaxation pseudopotential model with a modified force scheme is introduced and validated through the Laplace law and thermodynamic consistency. Subsequently, the near-wall bubble collapse evolution is simulated using the improved model, and the process of the bubble collapse evolution is obtained. The accuracy of the numerical simulation results is confirmed by comparing with previous experimental results. Based on the obtained flow field information, including velocity and pressure distributions, the dynamic behaviors during the bubble collapse are thoroughly analyzed. The results show that the micro-jets released during the near-wall bubble collapse primarily originate from the first collapse, while the shock waves are generated during both the first and the second collapse. Notably, the intensity of the shock waves produced during the second collapse is significantly higher than that during the first collapse. Furthermore, the distribution characteristics of pressure and velocity on the wall during the near-wall bubble collapse are analyzed, revealing the load mechanism of wall damage caused by bubble collapse. The results show that the wall is subjected to the combined effects of shock waves and micro-jets: shock waves cause large-area surface damage due to their extensive propagation range, whereas micro-jets lead to concentrated point damage with their localized high-velocity impact. In summary, this study elucidates the evolution of near-wall bubble collapse and the load mechanism of wall damage induced by bubble collapse, which provides theoretical support for further utilizing the cavitation effects and mitigation of cavitation-induced damage.
PHYSICS OF GASES, PLASMAS, AND ELECTRIC DISCHARGES
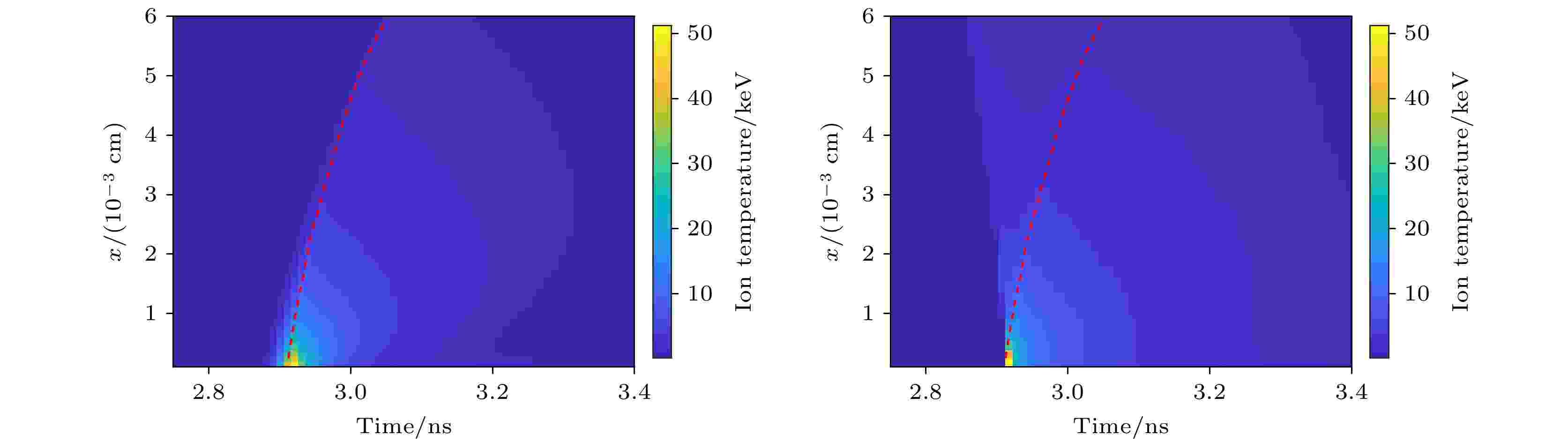
EDITOR'S SUGGESTION
2025, 74 (10): 105201.
doi: 10.7498/aps.74.20250018
Abstract +
CONDENSED MATTER: STRUCTURAL, MECHANICAL, AND THERMAL PROPERTIES
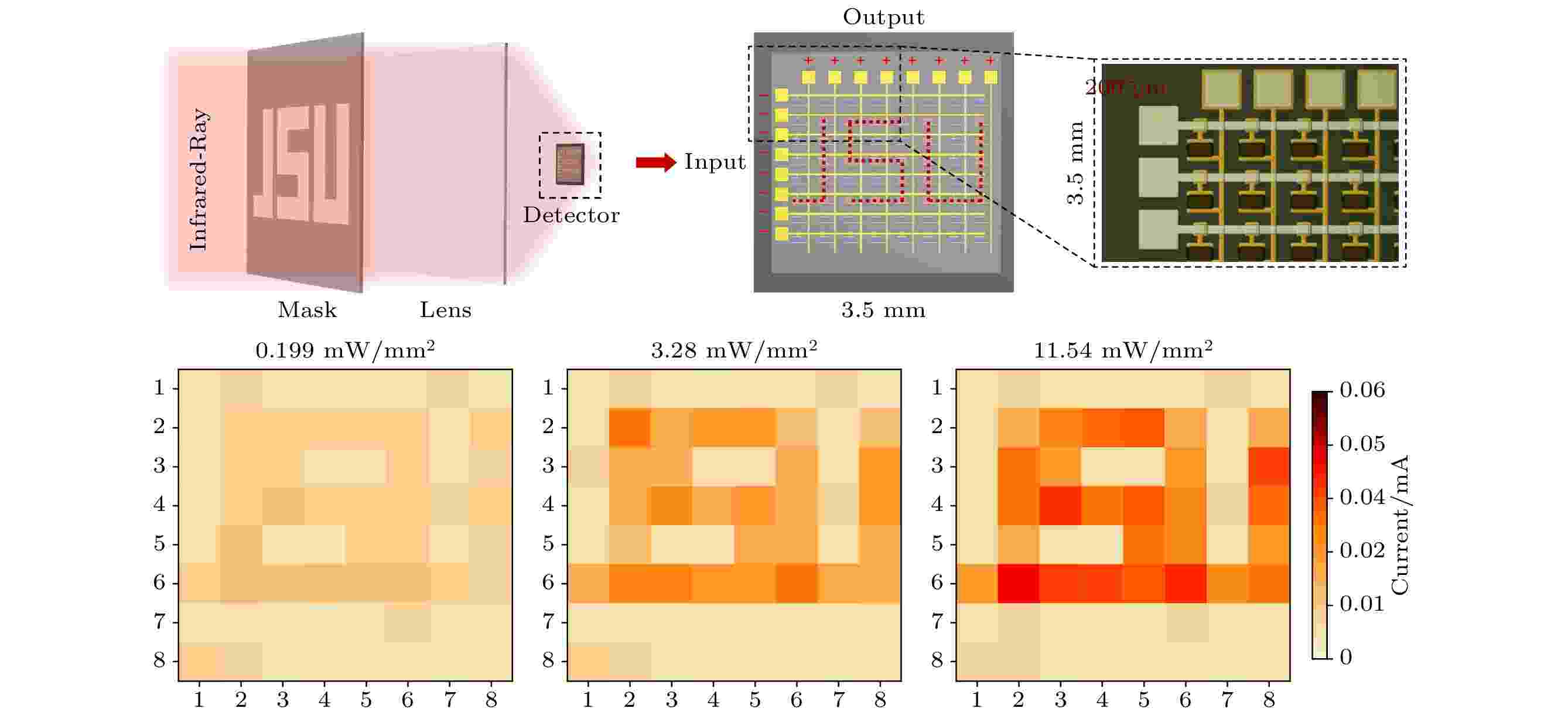
2025, 74 (10): 106101.
doi: 10.7498/aps.74.20241761
Abstract +
Infrared focal plane array (IRFPA) detector, a key research focus in next-generation infrared detection technology, plays a crucial role in optoelectronic sensing. Here is the report on the integration and reliability of a PbSe-based IRFPA employing a row-column scanning readout architecture. This design features a surface passivation layer and through-hole structures to ensure robust electrical connectivity, thereby enhancing both stability and performance. The detector, with dimensions of 3.5 mm × 3.5 mm, a pixel size of 200 μm × 100 μm, and a pixel pitch of 200 μm, demonstrates structural integrity validated by electro-thermal simulations. At room temperature, the pixel-level and imaging assessments reveal an average detectivity value of 9.86×109 Jones and a responsivity value of 1.03 A/W, with a 100% effective pixel yield. Remarkably, the device retains high stability, exhibiting only a 3.6% performance decline after 150-day air exposure, which is attributed to the protective effect of the passivation layer. Infrared imaging under different light intensities shows pronounced contrast, confirming the sensitivity of the detector to illumination gradients. These results provide critical technical insights and a theoretical framework for advancing high-performance, stable PbSe-based IRFPA detectors.
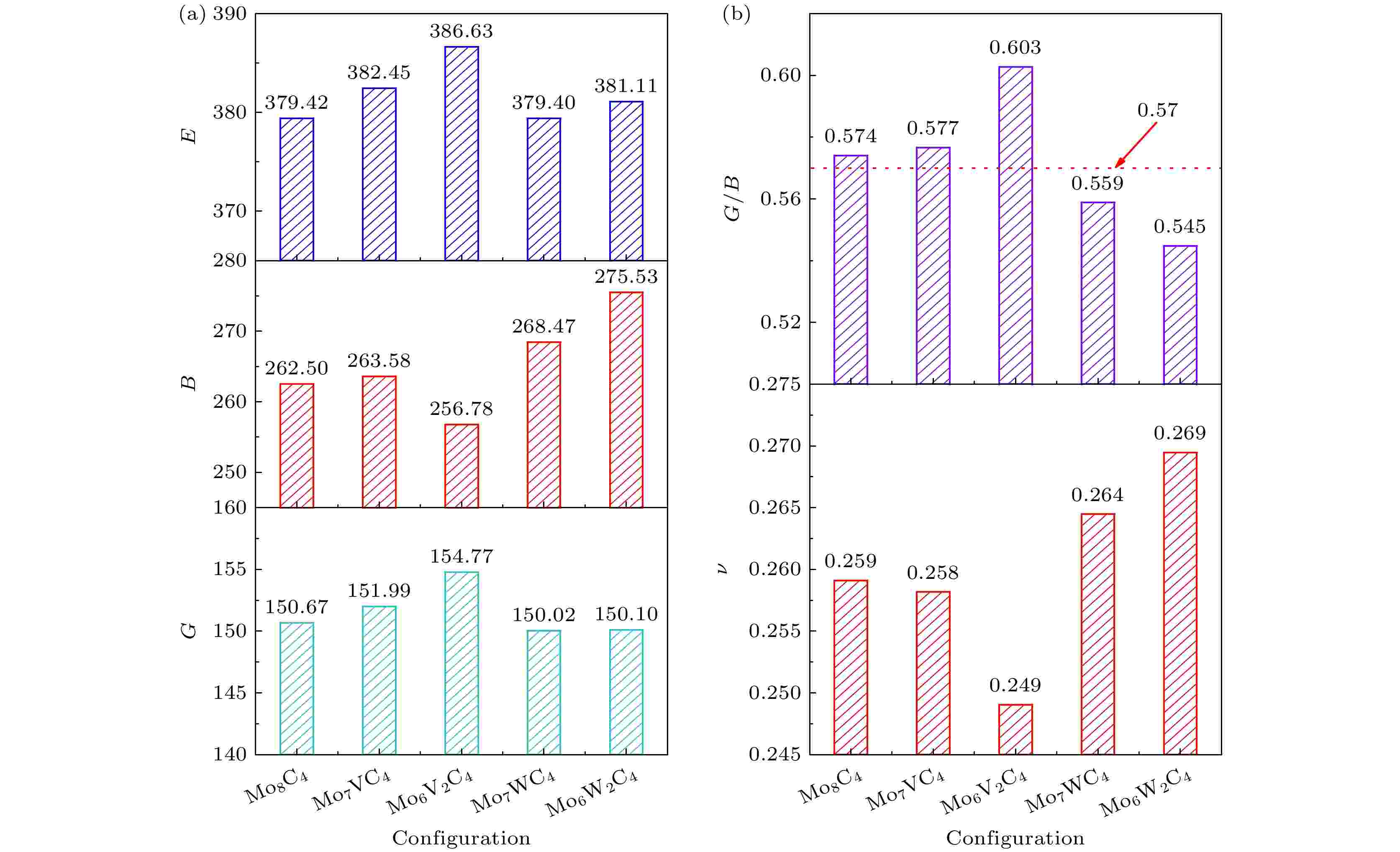
2025, 74 (10): 106301.
doi: 10.7498/aps.74.20250039
Abstract +
Secondary hardening ultra-high-strength steel is widely utilized in aerospace and other advanced engineering, with the nanoscale M2C precipitates serving as the primary strengthening factor. Mo plays a crucial role in the forming of Mo2C secondary hardening phase, which can form composite M2C precipitates with elements such as Cr, V, and W, thereby modifying the composition and properties of Mo2C. To investigate the effects of V and W doping on Mo2C, first-principles calculations are used to analyze the formation enthalpy, electronic structure, and mechanical properties of the doped systems. The CASTEP module is utilized in this study, with the Perdew-Burke-Ernzerhof (PBE) functional adopted in the generalized gradient approximation (GGA) framework. The results indicate that V doping reduces the lattice parameters and the formation enthalpy, thereby enhancing structural stability. In contrast, W doping increases the lattice parameters and lowers the formation enthalpy but leads the structural stability to decrease. In terms of mechanical properties, V doping reduces toughness while increasing hardness, whereas W doping improves the strength-toughness balance by mitigating the rate of hardness reduction. Covalent bonds are formed within the system, with V and W doping changing their characteristics: compared with the C—Mo bond, the C—V bond exhibits weaker covalency, while the C—W bond displays stronger covalency. Additionally, V doping enhances the stability of Mo—C bonds, whereas W doping reduces their stability. Charge population analysis reveals that metal atoms (Mo, V, and W) act as electron donors, while carbon atoms act as electron acceptors.
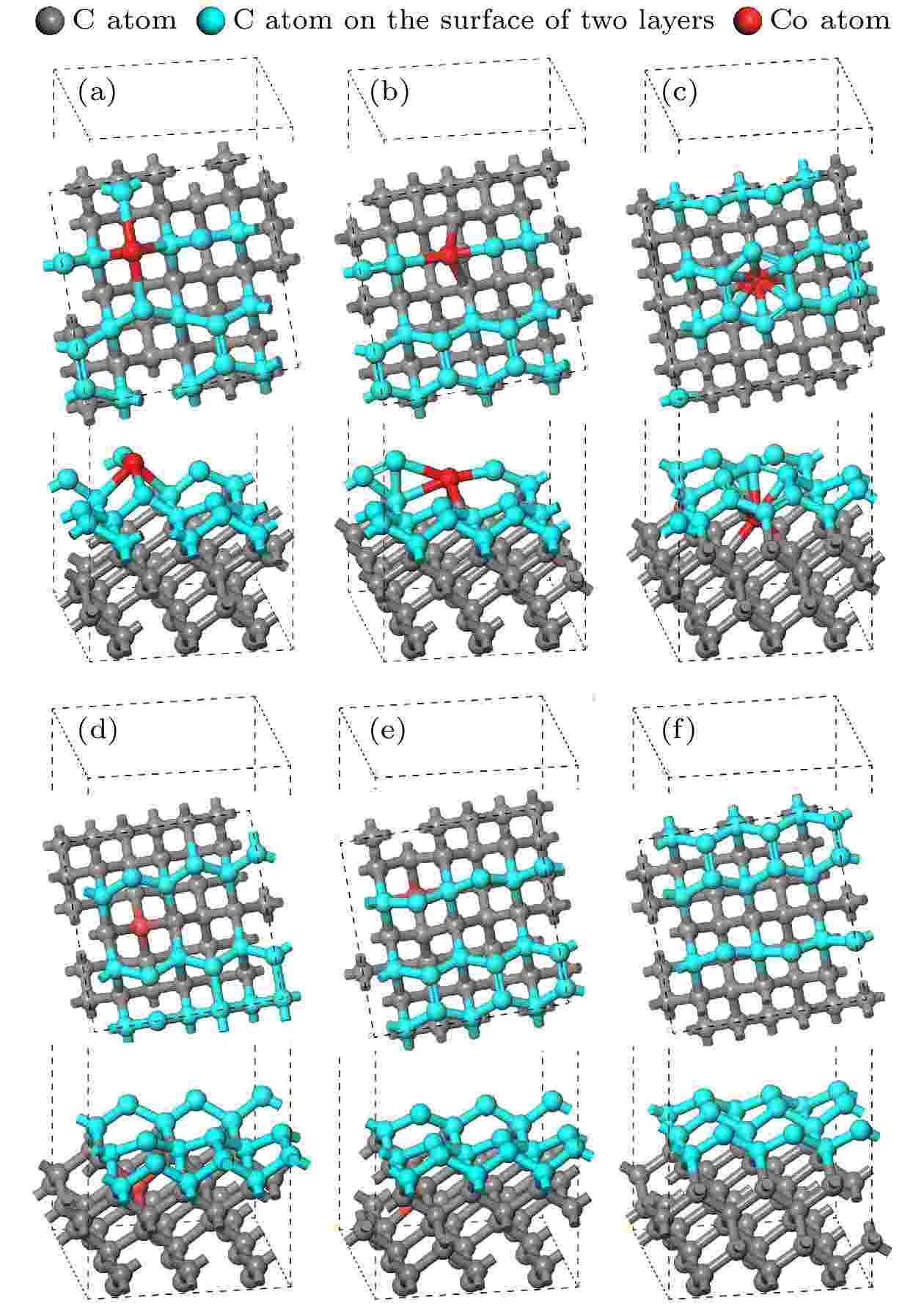
2025, 74 (10): 106701.
doi: 10.7498/aps.74.20250149
Abstract +
Diamond coating has many excellent properties such as extreme hardness, high elastic modulus, high thermal conductivity, low friction coefficient, low thermal expansion coefficient, and good corrosion resistance. Those properties are close to natural diamond’s, thereby making the diamond coating an ideal new type of wear-resistant tool coating material. However, a large number of experiments have proved that during the deposition of diamond coating, the bonding phase cobalt on the surface of impregnated diamond substrate will generate a layer of graphite at the interface, which seriously weakens the adhesive strength between the substrate and the coating. To thoroughly solve this problem, it is necessary to investigate the microscopic process of graphitization caused by the Co element embedded on the substrate surface. Therefore, the first principle theory is adopted to simulate and analyze the interfacial adhesive strength of diamond coating when Co atom is embedded at different depths on the surface of impregnated diamond substrate, thereby exploring the mechanism of the influence of bonding phase Co element in the substrate on the diamond coating and the mechanism of Co promoting diamond graphitization. The calculation results show that the interfacial binding energy first decreases and then increases with the increase of Co embedding depth in the substrate. When Co atom is embedded in the third layer, obvious graphite structures are prone to appear at the interface, and Co promotes diamond graphitization most significantly, resulting in the minimum bonding strength between the film and substrate interface. The results of structure and charge indicate that under the influence of surface effect and Co—C bond length, the C atoms in the second layer of the substrate move to the first layer and the hybridization mode changes from sp3 to sp2. Meanwhile, this movement leads to an increase in the interaction space and quantity between Co atoms and the surrounding C atoms. In addition, there are many unpaired electrons in the Co valence layer, which can easily mix and rearrange electron orbitals with the surrounding C atoms, ultimately resulting in a graphite structure on the substrate surface. When Co atoms are embedded in the fifth layer, the stable configuration of the substrate surface and the interfacial adhesive strength are no longer affected.
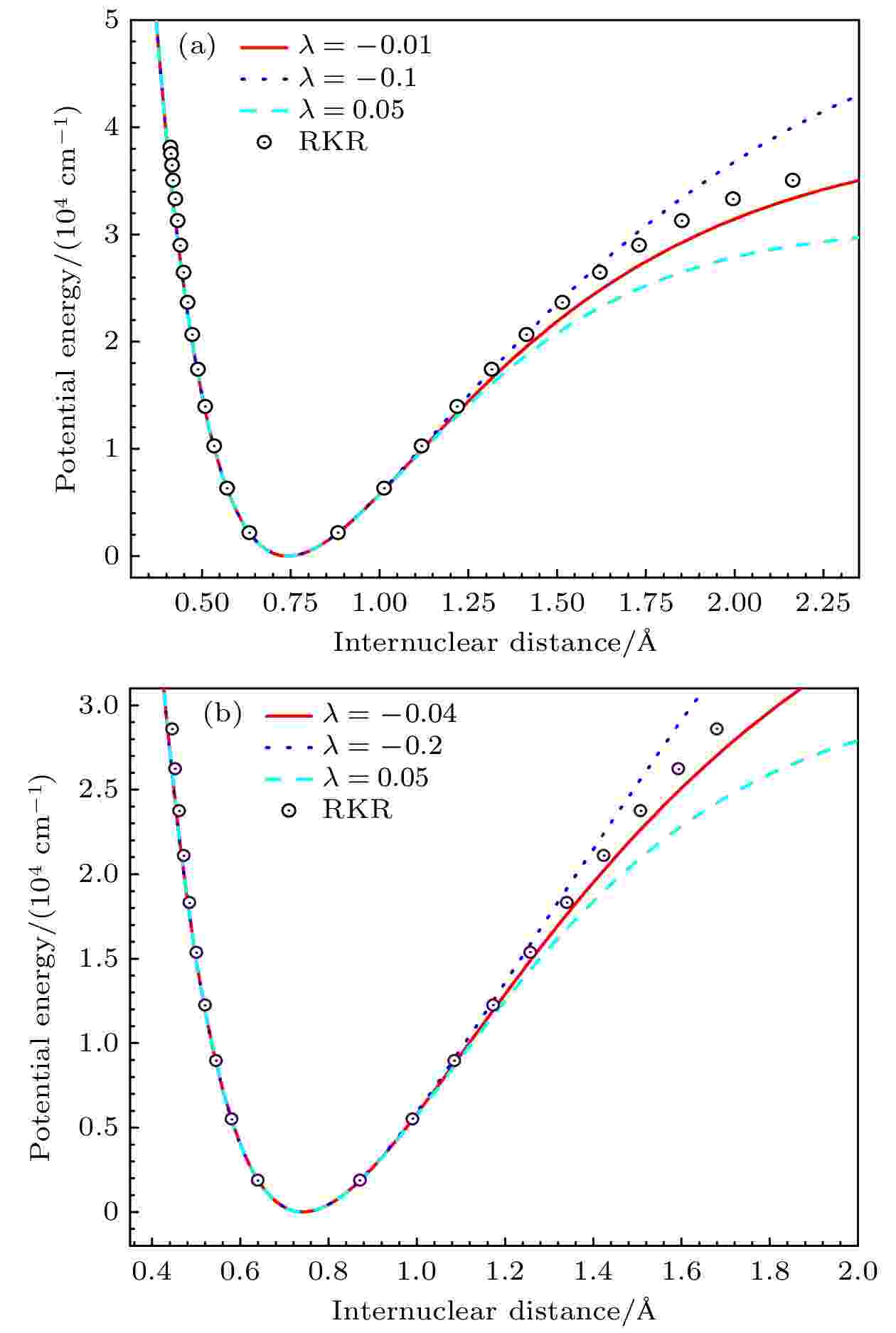
2025, 74 (10): 106702.
doi: 10.7498/aps.74.20241793
Abstract +
H2 molecule and their isotopes represent one of the modern clean energy sources. It is imperative to understand their thermodynamic properties for comprehending their behaviors under various conditions. Thereby promoting their more in-depth applications. In this paper, an extended improved multiparameter exponential-type potential (EIMPET) combined with the quantum statistical ensemble theory is used to investigate and analyze the thermodynamic properties of H2 and HD molecules. Firstly, reliable energy level data for molecules are obtained using the EIMPET potential. Subsequently, the one-dimensional Schrödinger equation is solved with the LEVEL program to determine the rovibrational energy levels of the molecules. Finally, the quantum statistical ensemble theory is integrated to determine the partition functions, molar heat capacity, molar entropy, molar enthalpy, and reduced molar Gibbs free energy of H2 and HD in a temperature range of 100–6000 K. The calculation results indicate that compared with IHH potential and IMPET potential, the EIMPET potential is closer to RKR data. A comparison of the calculated thermodynamic properties of the molecules reveals that the results from the EIMPET potential-based method accord well with those from the NIST database. Specifically, for H2, the root mean square (RMS) errors for $ {C_{\text{m}}}\left( T \right) $, $ {S_{\text{m}}}\left( T \right) $, $ {G_{\text{r}}}\left( T \right) $, and $ \Delta {H_{\text{r}}}\left( T \right) $are 0.6894 J·K–1·mol–1, 0.3824 J·K–1·mol–1, 0.1754 J·K–1·mol–1, and 0.9586 kJ·mol–1, respectively, while for HD, the RMS errors are 0.3431 J·K–1·mol–1, 0.1443 J·K–1·mol–1, 0.0495 J·K–1·mol–1, and 0.4863 kJ·mol–1, respectively. All of these results are superior to those obtained using IMPET potential, and to those obtained using IHH potential as a whole. These findings demonstrate the advantages and practical applications of the EIMPET potential in calculating the thermodynamic properties of diatomic gas molecules, providing a foundation for subsequently studying the thermodynamic properties of triatomic molecules.
CONDENSED MATTER: ELECTRONIC STRUCTURE, ELECTRICAL, MAGNETIC, AND OPTICAL PROPERTIES
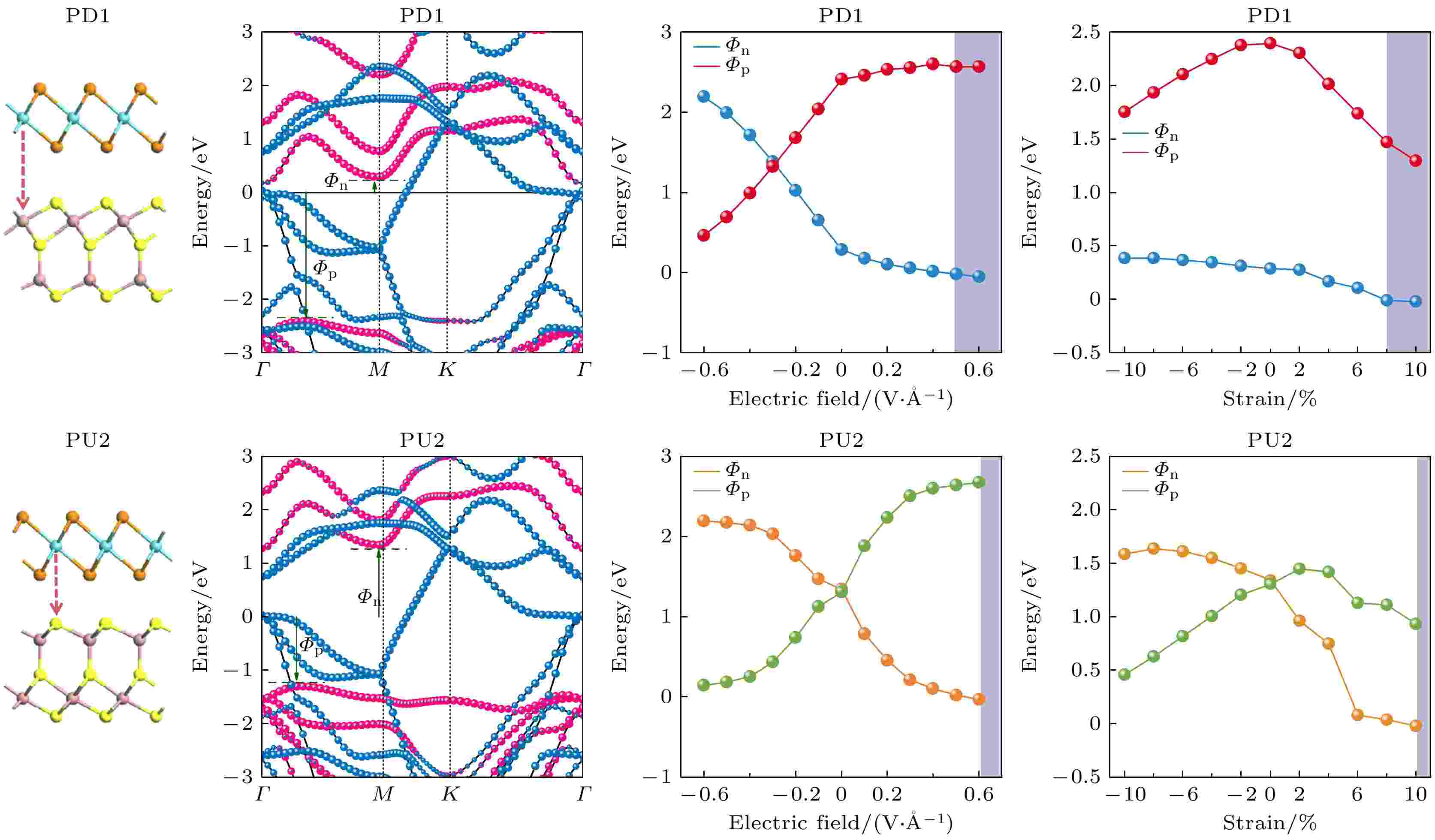
2025, 74 (10): 107101.
doi: 10.7498/aps.74.20241705
Abstract +
A monolayer ferroelectric semiconductor, Ga2S3, has received extensive attention because of its outstanding ductility, extremely high carrier mobility and unique out-of-plane asymmetric polarization characteristics. In this work, T-NbTe2/Ga2S3 ferroelectric heterojunctions are constructed using out-of-plane asymmetric polarization characteristics of Ga2S3. The structural stability, preparation possibility and electrical contact properties for various ferroelectric heterojunction T-NbTe2/Ga2S3 ferroelectric heterojunctions with the different polarization directions of Ga2S3 are systematically studied by the first-principles calculations. It is found that heterojunctions T-NbTe2/Ga2S3 exhibit sensitive responses to out-of-plane asymmetric polarization characteristics of Ga2S3. The two heterojunctions with the most stable energy, PD1 ($ \boldsymbol P_{\downarrow}$) and PU2 ($ \boldsymbol P_{\uparrow} $), in the intrinsic state form N-type and P-type Schottky contact, respectively. The polarization characteristics of the ferroelectric semiconductor Ga2S3 are dependent on the contact type of the Schottky barrier in the ferroelectric heterojunction T-NbTe2/Ga2S3, which provides a practical approach for designing multifunctional Schottky devices. Specifically, the electrical contact depends on the external electric field. For the heterojunction, PD1 (and PU2), the contact can transition from Schottky contact to Ohmic contact at an electric field strength of +0.5 V/Å (+0.6 V/Å). Besides electric field, the contact properties of both heterojunctions PD1 and PU2 may also be tuned by an external biaxial strain. For the heterojunction, PD1, the contact can transition from Schottky contact to Ohmic contact at a biaxial strain tensile of 8%. And for the heterojunction, PU2, the contact can transition from P-type Schottky contact to N-type Schottky contact at a biaxial strain tensile of 2%, then from N-type Schottky contact to Ohmic contact at a strain tensile of 10%. These results provide a theoretical reference for designing two-dimensional ferroelectric nanodevices with high-performance electrical contact interfaces.
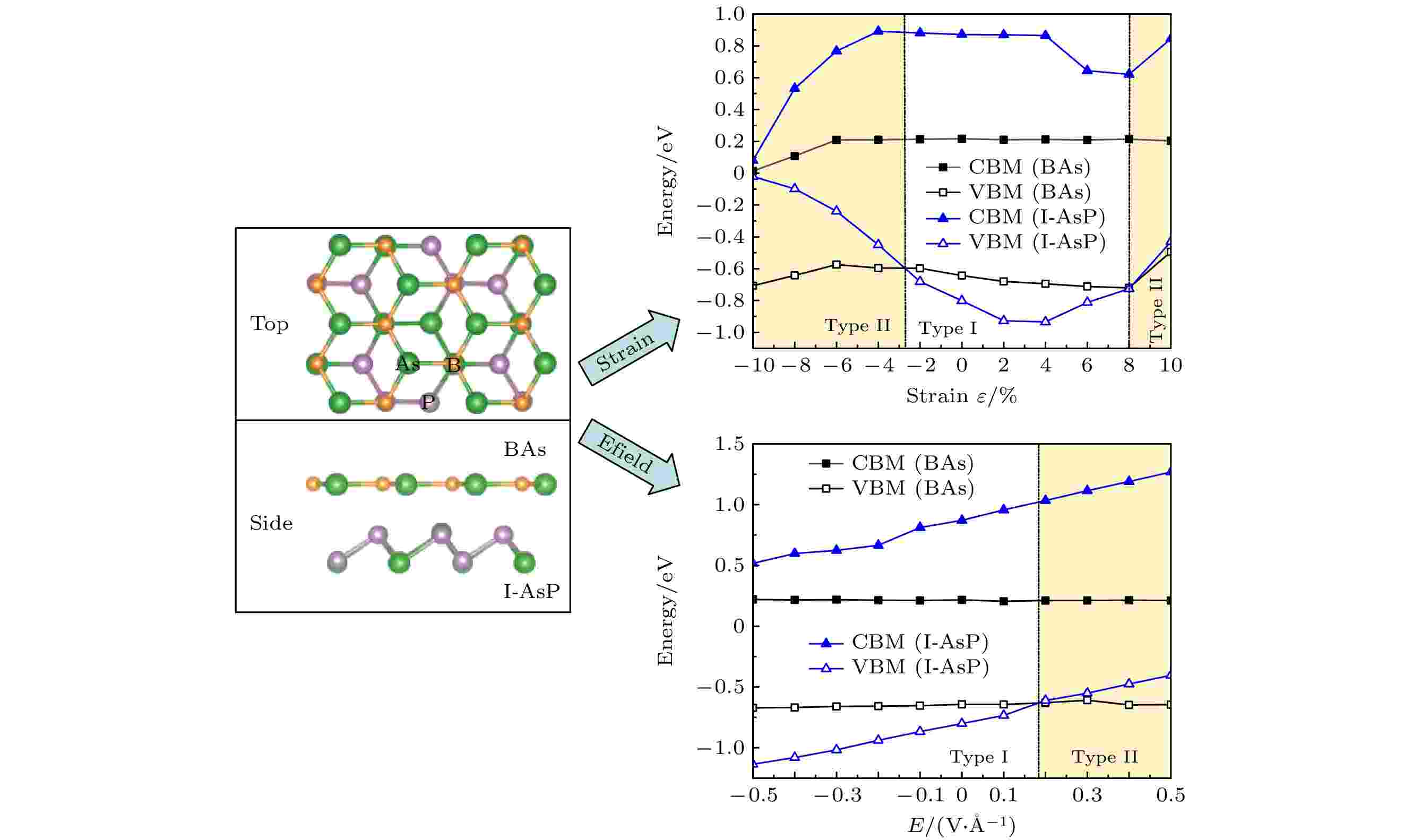
2025, 74 (10): 107102.
doi: 10.7498/aps.74.20250196
Abstract +
In recent years, two-dimensional (2D) materials have attracted considerable attention due to their outstanding optical and electronic properties, and they have shown great potential applications in next-generation solar cells and other optoelectronic devices. In this work, density functional theory (DFT) is used to systematically study the electronic and optoelectronic properties of the heterojunction formed by 2D BAs and I-AsP monolayers, as well as the response of this heterojunction under biaxial strain and electric field. The calculation results show that in the ground state, the four vertically stacked BAs/I-AsP heterostructures all have stable geometric structures, and their band gaps range from 0.63 to 0.86 eV. Compared with their constituent monolayers, these heterostructures have the increased optical absorption coefficients (the absorption coefficient in the x-direction reaches 106 cm–1), and they can effectively separate the photogenerated electron-hole pairs. Of the four structures, the A1 structure exhibits the smallest interlayer spacing, the smallest binding energy, and the highest stability. It has a type-I band alignment and a structure of a direct-band-gap semiconductor with band gaps of 0.86 eV (PBE) and 1.26 eV (HSE06), which can be used in the field of light-emitting diodes. The band gap and band type of the heterostructure can be effectively changed by applying biaxial strain and electric field. Under the application of biaxial tensile or compressive strain in a range of –10% to 8%, the band gap increases accordingly. When the tensile strain is greater than 8%, the band gap starts to decrease. When the biaxial strain ε ≤ –3% and ε > 8%, the heterojunction transitions from a type-I band alignment to a type-II band alignment. Under tensile strain, the absorption spectrum undergoes a red shift, while compressive strain leads to a blue shift of the absorption spectrum. Similarly, the externally applied electric field linearly affects the band gap of the BAs/I-AsP heterojunction in a range from –0.5 to 0.5 V/Å, and the band gap decreases as the electric field increases. When a positive electric field with E ≥ 0.2 V/Å is applied, the band alignment of the heterojunction can also transition from type-I to type-II. The BAs/I-AsP heterojunction has strong absorption properties in the ultraviolet and visible light ranges. Based on the Scharber model, the theoretical power conversion efficiency (PCE) η of the BAs/I-AsP heterojunction is found to be greater than 13%, which is higher than those of 2D heterojunction materials such as Cs3Sb2I9/InSe (η = 3.3%), SiPGaS/As (η = 7.3%) and SnSe/SnS (η = 9.1%). This further expands the application scope of the BAs/I-AsP heterojunction, making it expected to play an important role in the field of photodetectors and solar cells.
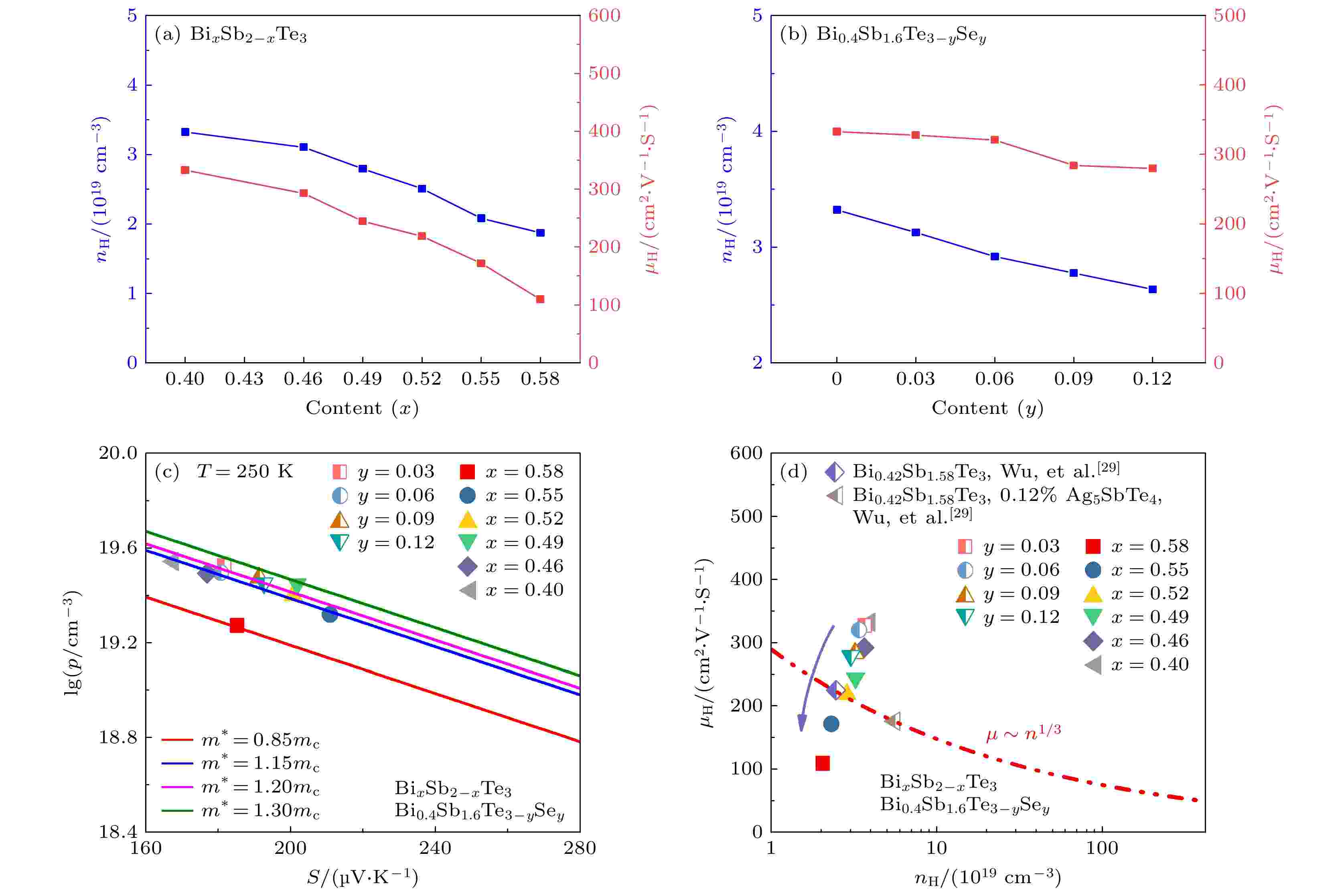
EDITOR'S SUGGESTION
2025, 74 (10): 107103.
doi: 10.7498/aps.74.20250150
Abstract +
Bi2Te3-based compounds are the thermoelectric materials available only commercially, but the research on their low-temperature performances below 300 K are still insufficient. The influences of Bi/Sb ratio modulation and Se substitution on the electrical and thermal transport properties of BixSb2–xTe3 and Bi0.4Sb1.6Te3–ySey materials are systematically investigated in this work, aiming to optimize their thermoelectric performance in cryogenic regions through combined bandgap tuning and defect engineering. Materials are synthesized using a melt-quenching and spark plasma sintering process, and then phase analysis is conducted via X-ray diffraction and microstructural characterization by electron probe microanalysis. First-principles calculations and Hall effect measurements are used to investigate their defect formation mechanisms and carrier transport behaviors. In the BixSb2–xTe3 system, the increase of Bi content reduces the bandgap from 0.168 eV for Bi0.4Sb1.6Te3 to 0.113 eV for Bi0.58Sb1.42Te3, shifting the peak ZT temperature to lower ranges. However, the enhancement of alloy scattering leads the carrier mobility to decrease from 332 to 109 cm2/(V·s) and power factor to fall from 4.58 to 1.12 mW/(m·K2). To solve this problem, Se is substituted for the Te lattice of Bi0.4Sb1.6Te3. First-principles calculations reveal that the Se substitution reduces the formation energy of SeTe + BiSb complex, thus effectively suppressing SbTe antisite defects. This will result in the carrier concentration decreasing from 3.32×1019 to 2.64×1019 cm–3 while maintaining high mobility at 279 cm2/(V·s). Concurrently, Se-induced point defects enhance phonon scattering, reducing lattice thermal conductivity from 0.46 to 0.38 W/(m·K), a decrease of 17%. Bi0.4Sb1.6Te2.97Se0.03 sample achieves a ZT value of 0.93 at 220 K, which is 16% higher than the pristine Bi0.4Sb1.6Te3 sample with a ZT value of 0.80. The peak ZT increases from 1.17 to 1.31 at 350 K, an increase of 12%. These improvements arise from the synergistic effects of band engineering, where flattened valence band edges increase effective mass, and defect engineering, where antisite defects and strengthens phonon scattering are suppressed. This work provides a dual optimization strategy for BiSbTe-based materials, i.e. balancing bandgap reduction by controlling defects to improve cryogenic performance. The findings are particularly significant for the applications of BiSbTe-based materials in infrared detectors and multistage thermoelectric cooling systems.
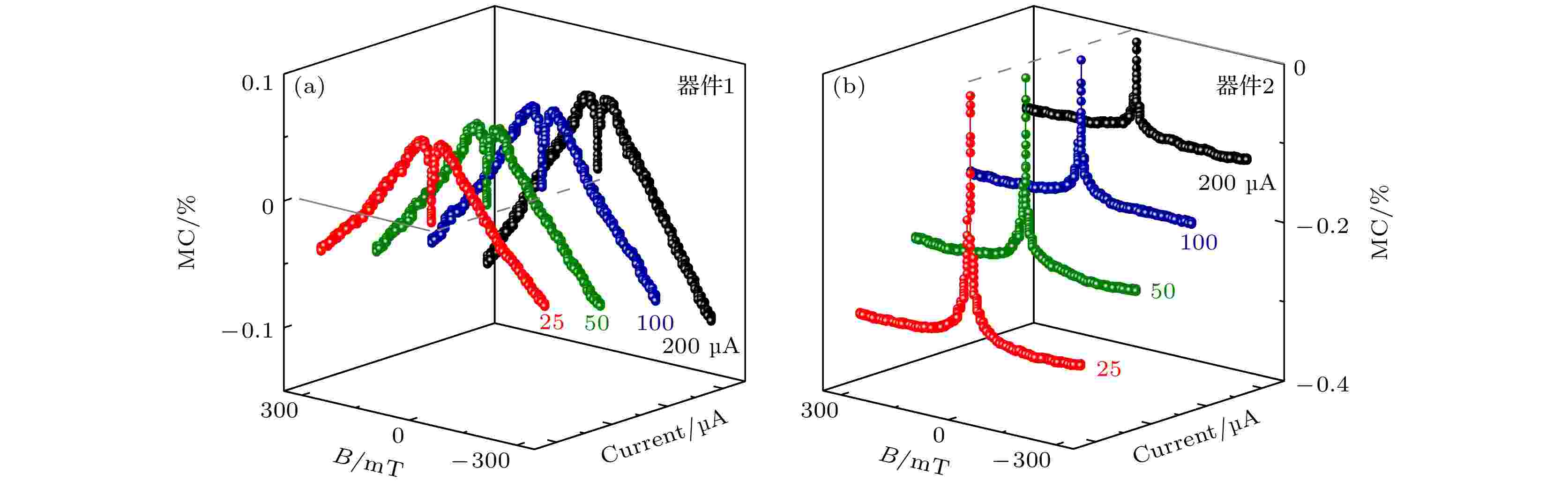
EDITOR'S SUGGESTION
2025, 74 (10): 107201.
doi: 10.7498/aps.74.20241601
Abstract +
Charge balances can influence the emission efficiency of exciplex-based organic light-emitting diodes (OLEDs), but so far, the physical mechanism behind this phenomenon is not fully understood. Here, organic magnetic field effects (OMFEs) including magneto-conductance (MC), magneto-electroluminescence (MEL), and magneto-efficiency (Mη) are used as fingerprint probing tools to study physical mechanism of influence of charge balance on the emission efficiency of exciplex-based OLEDs. Specifically, low- and high-field effects of MC traces [MCL (|B| ≤ 10 mT) and MCH (10 < |B| ≤ 300 mT)] from the unbalanced device are separately attributed to the magnetic field (B)-mediated intersystem crossing (ISC) process and the B-mediated triplet-charge annihilation (TCA) process between triplet exciplex states and excessive charge carriers, whereas those from the balanced device are respectively attributed to the B-mediated reverse intersystem crossing (RISC) process and the balanced carrier injection. As the injection current decreases from 200 to 25 μA, low-field effects of MEL traces (MELL) form the unbalanced device always reflect the B-mediated ISC process, but those from the balanced device exhibit a conversion from ISC process to RISC process. Furthermore, although low-field effects of Mη traces (MηL) from unbalanced device and balanced device are attributed to the B-mediated ISC process, MηL value in the balanced device is approximately one-fourth of that in the unbalanced device. These different MC, MEL, and Mη traces reveal that the balanced carrier injection can increase the number of triplet exciplex states via weakening the TCA process, which leads to the enhanced RISC process. Because RISC can convert dark triplet exciplex states into bright singlet exciplex states, the emission efficiency of the balanced device is higher than that of the unbalanced one. Obviously, in this work OMFEs are used to provide a new physical mechanism for charge balance that influences the emission efficiency of exciplex-based OLEDs.
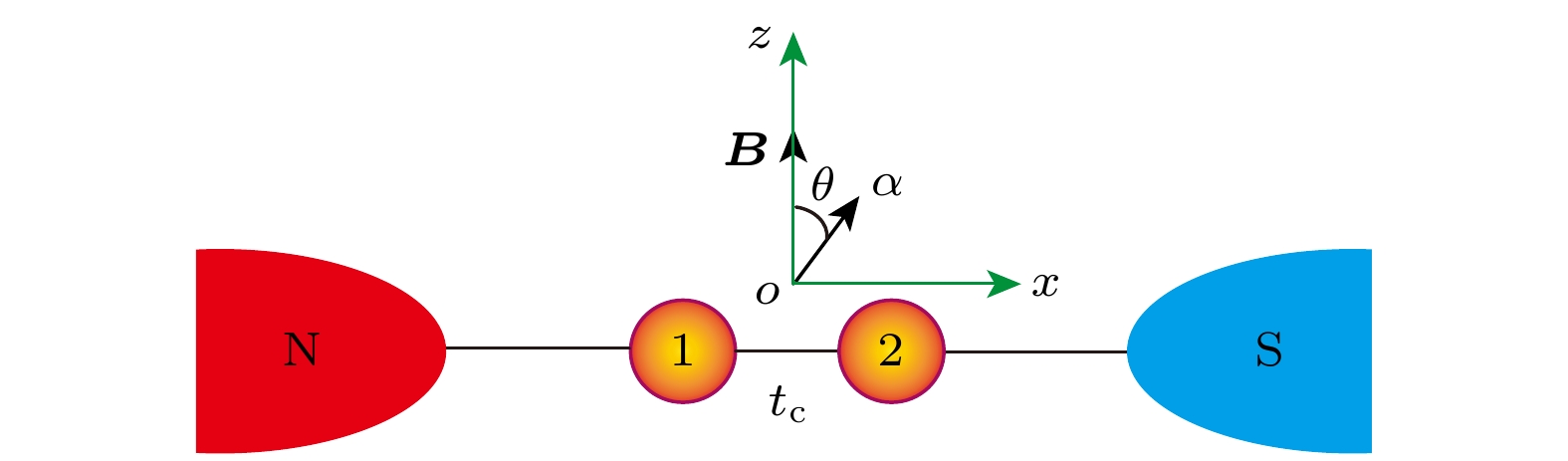
EDITOR'S SUGGESTION
2025, 74 (10): 107301.
doi: 10.7498/aps.74.20241756
Abstract +
The normal metal-quantum dots-superconductor hybrid system is a good platform for studying the mechanism of thermoelectric conversion. In terms of non-equilibrium Keldysh Green’s function formalism and linear response theory, the charge and spin thermoelectric transport characteristics of a normal-double quantum dot-superconductor hybrid system with spin-orbit coupling are studied in this work. We delve into the relationship between thermoelectric coefficients and the system parameters, and find both charge and spin thermoelectric coefficients exhibit distinct symmetry in the parameter space composed of temperature and energy. The increase in temperature leads to a decrease in conductance within the energy gap, which is attributed to the reduction in Andreev transport. However, outside the energy gap, the conductance gradually increases, and the thermal conductance is gradually enhanced. This is because more quasiparticles outside the energy gap participate in thermoelectric transport, and a large charge thermopower is generated in the region far from the energy gap. It is found that the thermoelectric figure of merit is greater than 1, indicating a strong violation of the Wiedemann-Franz law. With the increase of temperature, the large spin thermopower as well as spin thermoelectric figure of merit can be obtained outside the energy gap. The charge (spin) thermopower and the thermoelectric figure of merit show the rich evolutionary characteristics as functions of energy level and Zeeman energy. With the disappearance of the charge thermopower, the spin thermopower still has a finite value, which leads to the emergence of a pure spin Seebeck effect. This is helpful for designing a pure spin current thermoelectric generator. Due to a competitive mechanism between the spin-orbit coupling effect and the Zeeman field, thermoelectric coefficients decrease with the strength of spin-orbit interaction increasing, but one still can obtain the spin thermoelectric quantities which meet the practical needs by regulating the strength of spin-orbit coupling and the Zeeman energy. The evolution pattern of the thermoelectric coefficientss in the energy space indicates that the enhancement of thermoelectric conversion efficiency can be achieved by modulating the energy levels of double quantum dots. In addition, this hybrid system can act as a heat engine to achieve the conversion of heat into work. Although its power and efficiency do not evolve synchronously, thermodynamic performance that meets practical needs can still be obtained in certain parameter regions. The research results of this work hold theoretical and practical significance for understanding the thermoelectric transport and thermodynamic performance of hybrid thermoelectric systems.
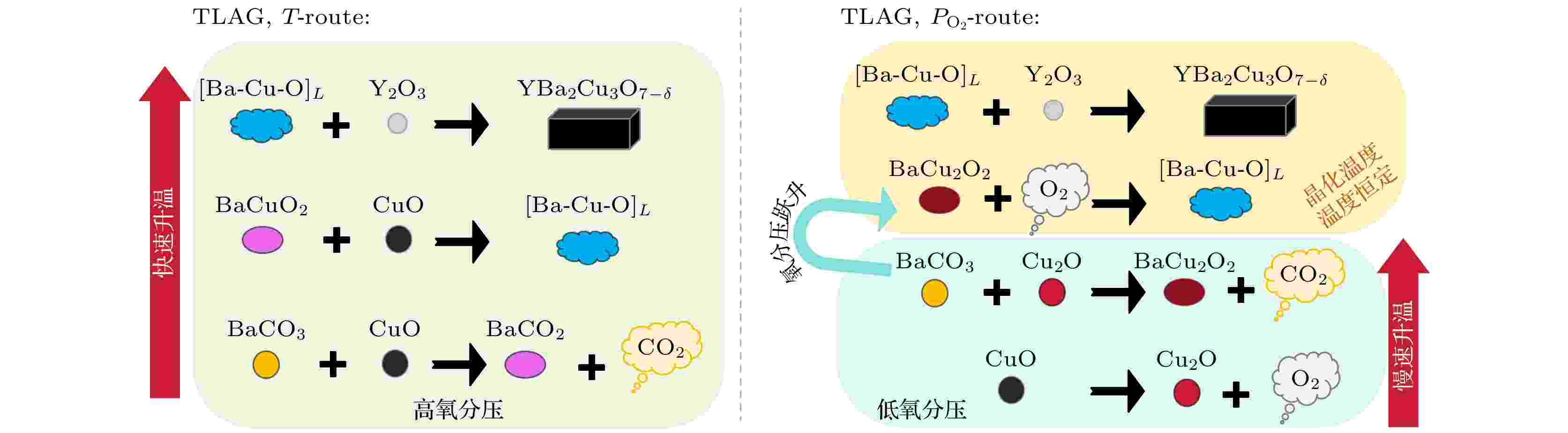
EDITOR'S SUGGESTION
2025, 74 (10): 107401.
doi: 10.7498/aps.74.20250037
Abstract +
The epitaxial orientation of YBa2Cu3O7–δ grown via the oxygen partial pressure jump pathway in transient liquid-phase assisted chemical solution deposition (TLAG-CSD) depends on the barium-to-copper ratio in the precursor phase. To explore the mechanism behind this phenomenon, in this work we investigate the effects of different oxygen partial pressures and barium-to-copper ratio components on the barium-copper-oxygen liquid phase ([Ba-Cu-O]L) and the intermediate phase transition in the medium-high temperature heat treatment process. The research shows that the formation of the liquid phase exhibits a point-to-surface characteristic; the temperature and morphological differences in the liquid phase are mainly determined by the composition, with oxygen partial pressure only playing a supporting role. Y∶Ba∶Cu = 0∶3∶7 (0-3-7) components all appear before Y∶Ba∶Cu = 0∶2∶3 (0-2-3) components in the liquid phase, with a temperature difference of 20 ℃ (high oxygen partial pressure) or 40 ℃ (low oxygen partial pressure). Experimental results indicate that there are differences in the intermediate phase properties between these two components. Under high oxygen partial pressure, the intermediate phase BaCuO2 exhibits a single characteristic peak in the 0-3-7 component, with large and dispersed grains; the 0-2-3 component has multiple characteristic peaks, with small and dense grains. The surface area of the liquid phase region in the 0-3-7 component is smaller than that in the 0-2-3 component, resulting in different supersaturation levels of Y3+ in the liquid phases of the two components and causing orientation differences in YBCO. Finally, the basic model for the formation of fluorine-free liquid phase is summarized, and the complete [Ba-Cu-O]L film can be generated from the 0-2-3 component at high oxygen partial pressure and 750 ℃.
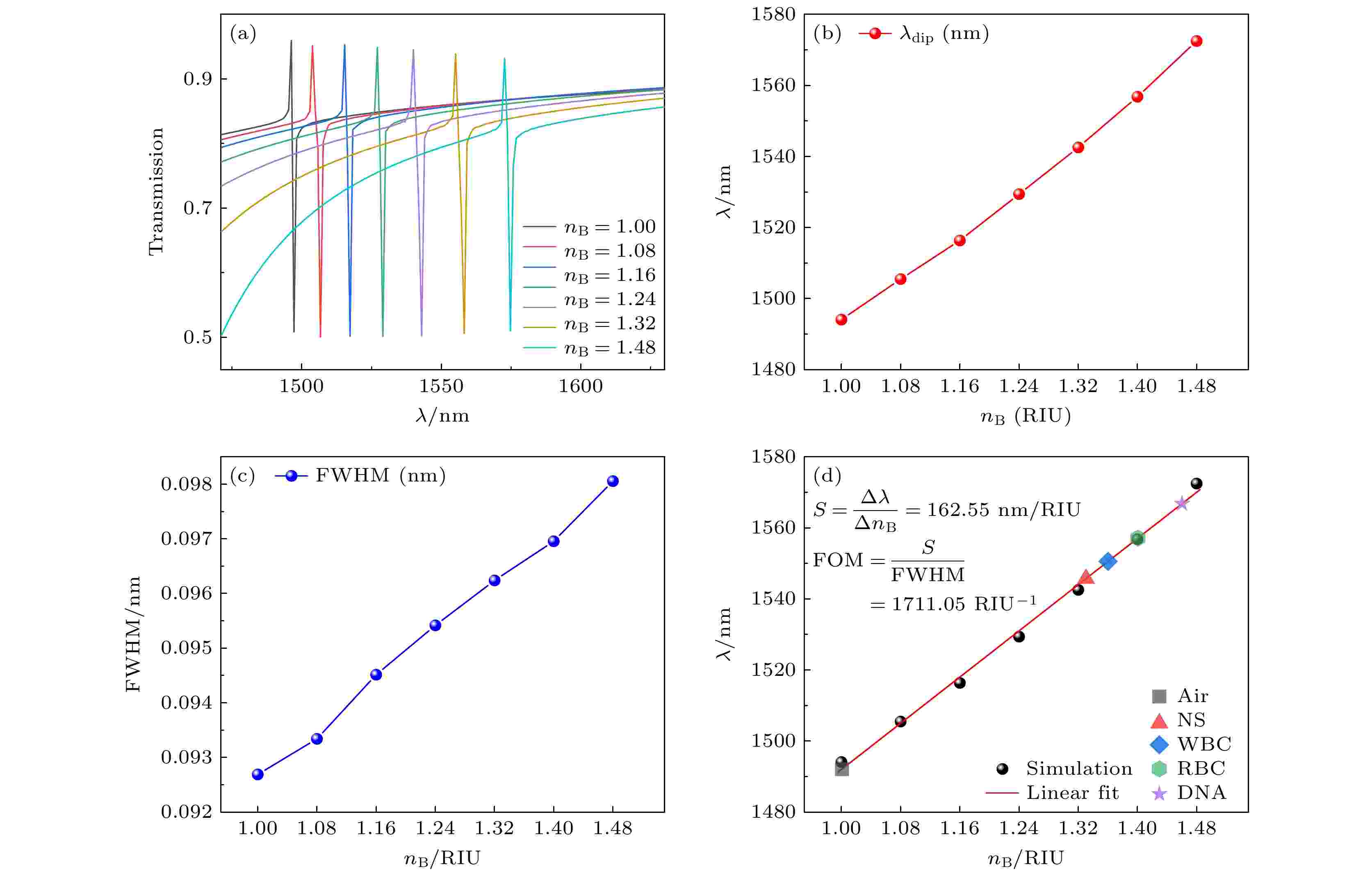
EDITOR'S SUGGESTION
2025, 74 (10): 107801.
doi: 10.7498/aps.74.20241752
Abstract +
In recent years, bound states in the continuum (BICs) have become a hot research topic because of their strong ability to facilitate light-matter interactions, and they are also an ideal platform for realizing optical resonances with ultra-high quality factors (Q). Nowadays, BICs have been found to exist in various photonic microstructures and nanostructures such as waveguides, gratings, and metasurfaces, among which metasurfaces have attracted much attention due to their ease of adjustment and considerable robustness. Traditional precious metal-based metasurfaces inevitably have low Q-factors due to the inherent defect of high ohmic losses. In contrast, due to lower ohmic losses, all-dielectric metasurfaces can be an excellent alternative to metallic metasurface structures. In this work, an all-dielectric metasurface is designed, with a silicon disc as the unit cell, and symmetric protected BIC (SP-BIC) is observed on the metasurface. When introducing eccentric holes to break the symmetry in the structural plane (QBIC), the SP-BIC can be transformed into a quasi-BIC, with radiation dominated by magnetic dipoles and has a high-quality Q-factor. For QBICs formed on the metasurface, the resonance wavelength is usually greatly dependent on the refractive index of the surroundings due to the strong localization of the electric field within the cell. As the refractive index of the background changes, the positions of the resonance peaks change accordingly, and identification sensing of some biological components is achieved by this principle. This metasurface-based bio-refractive index sensor is less invasive in free space and is expected to overcome the drawbacks of traditional electrochemical-based biosensing technologies, which have cumbersome detection steps and high time and material costs. In terms of sensing parameters, due to the quadratic inverse relationship between the quality factor and asymmetric parameters, by adjusting the asymmetric parameters, the quality factor will also change, thereby enhancing and adjusting the sensing performance. After adjusting, the refractive index sensing sensitivity and figure of merit of this metasurface reach 162.55 nm/RIU and 1711.05 RIU–1, respectively, which are higher than those achieved in many other existing studies. This high Q-factor all-dielectric metasurface design provides a new avenue for achieving high-sensitivity and high-precision bio-detection.
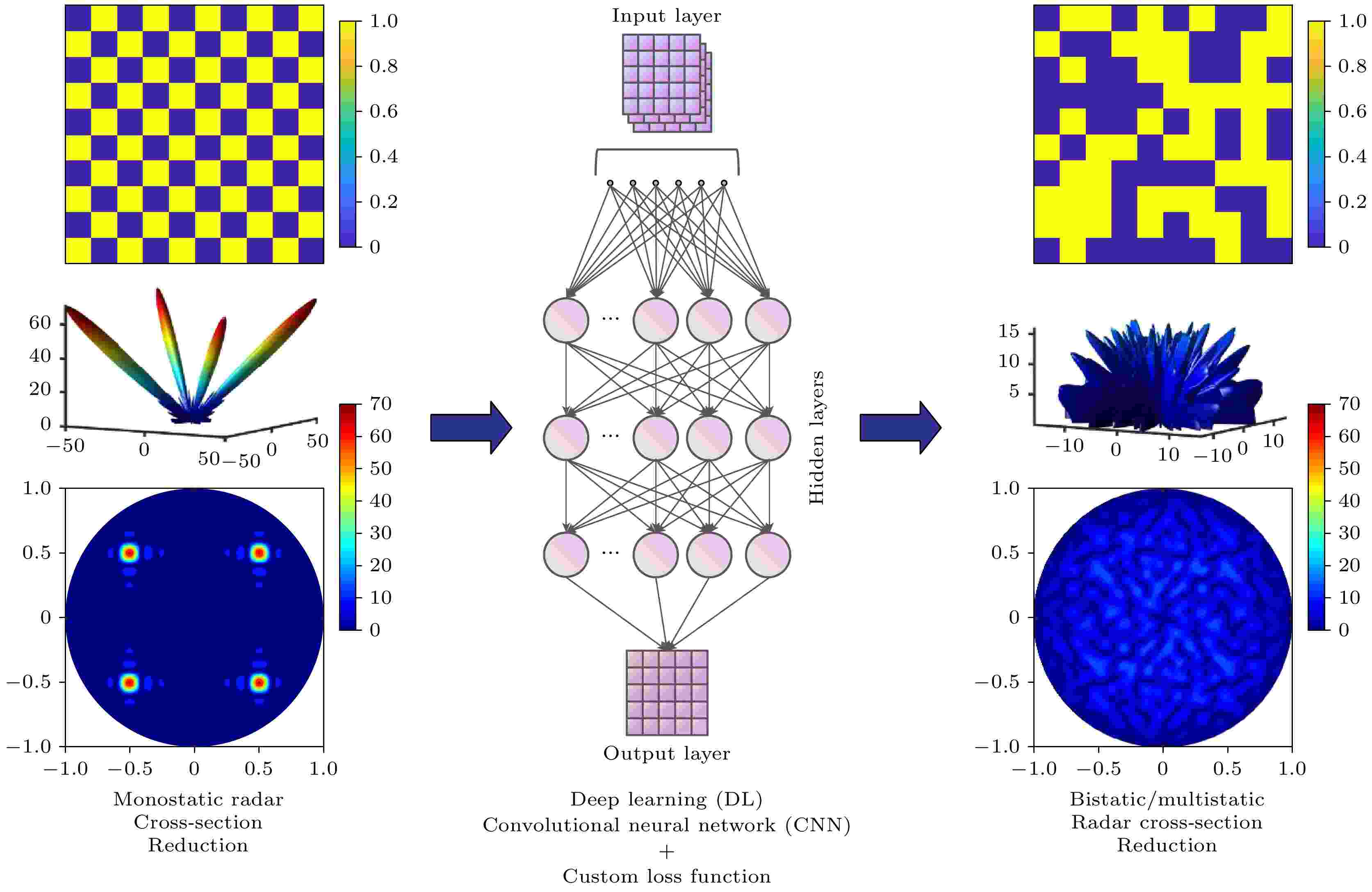
EDITOR'S SUGGESTION
2025, 74 (10): 107802.
doi: 10.7498/aps.74.20250109
Abstract +
Radar cross section (RCS), a crucial physical quantity that characterizes the backscattering intensity of targets under radar illumination, is the primary metric for assessing stealth capabilities. With the development of radar detection technologies, RCS reduction has become a forefront research topic in radar stealth, aiming to minimize target detectability. With the maturity of radar networking technology, the bistatic radar RCS reduction is becoming increasingly important in future electromagnetic stealth countermeasures compared with the monostatic radar RCS reduction. Artificial electromagnetic metasurfaces have introduced innovative technical approaches for realizing the bistatic radar RCS reduction. However, current metasurface designs still face challenges related to inefficiency and suboptimal performance, mainly due to the time-consuming nature of large-scale array optimization and the global extremum characteristics of bistatic radar RCS reduction. To overcome these limitations, this study proposes a few-shot convolutional neural network (CNN)-based approach, which achieves uniform full-space radar echo scattering by directionally optimizing metasurface phase distributions, thereby enabling effective bistatic radar RCS reduction. This approach integrates convolutional feature extraction, residual enhancement, and fully connected optimization modules with a customized loss function to efficiently capture the complex multidimensional relationships between diffuse reflection phases and the full-space RCS extrema. Theoretical calculations, full-wave simulations, and experimental tests show that the metasurface designed with this approach can achieve over 10 dB of Bistatic Radar RCS reduction in a frequency range from 7.26 GHz to 10.74 GHz. The method also ensures uniform diffuse reflection across the full space for various incidence angles (30°, 45°, 60°). Compared with traditional optimization algorithms, this method enhances RCS reduction by 17.2% while significantly improving computational efficiency. This approach provides a promising new technical paradigm for achieving full-space electromagnetic stealth in advanced weapon systems.
INTERDISCIPLINARY PHYSICS AND RELATED AREAS OF SCIENCE AND TECHNOLOGY
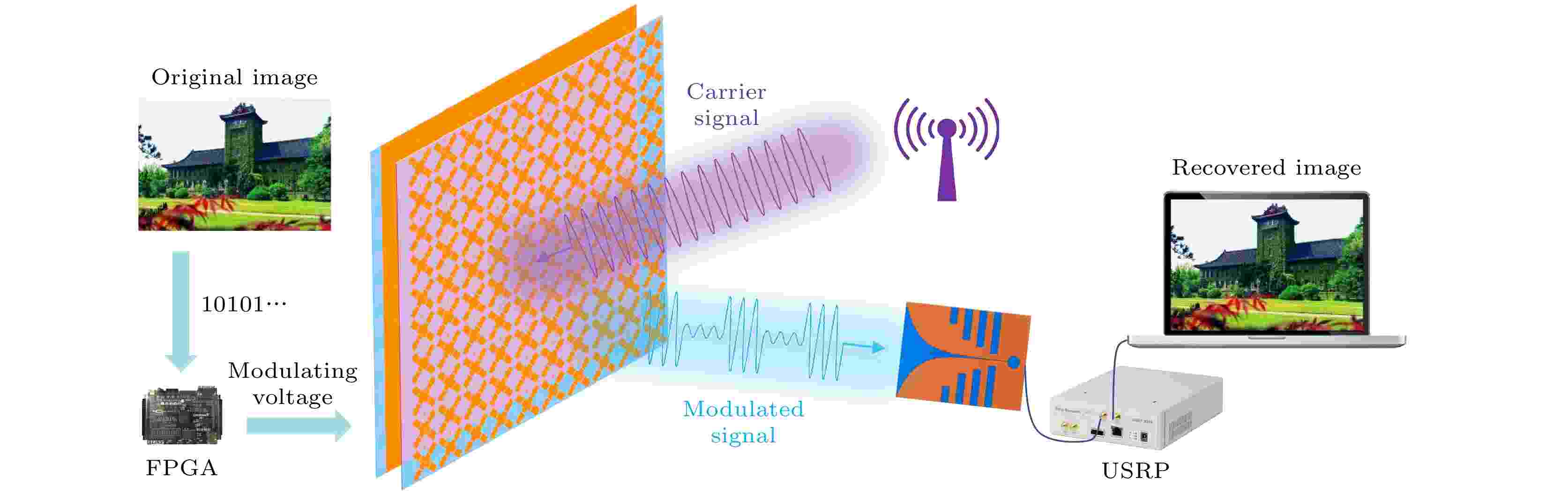
EDITOR'S SUGGESTION
2025, 74 (10): 108101.
doi: 10.7498/aps.74.20241668
Abstract +
In this paper, a method of designing broadband reconfigurable polarization-converting metasurface operating in L-band is proposed. This method can also be used to directly modulate the information by using two modulation modes: binary amplitude shift keying (BASK) and binary phase shift keying (BPSK). Switching the ON/OFF state of PIN diode can be used to modify the amplitude and phase responses of the cross-polarized reflection of the element in a frequency band of 1.17–1.66 GHz, thereby creating a 1-bit digital coding meta-atom. By changing the real-time coding patterns of amplitude and phase, the reconfigurable metasurface can control beams and information modulation. Simulation results show that by changing the coding patterns of the metasurface, twin-beams and four-beams with different reflection angles can be obtained which fully validates the control ability of dynamic far-field beam. As an experimental verification, a reconfigurable metasurface consisting of 10×10 meta-atoms is fabricated, and its beam control and information modulation functions are tested. The far-field patterns of the metasurface with different coding phase distributions are measured. Furthermore, modulation signals of varying high/low voltage levels and rates are loaded onto the metasurface, in order to control its modulation mode and rate. The modulated signals reflected from metasurface are captured by a high-speed radio-frequency (RF) oscilloscope at varying rates and reflection angles, and then demodulated so as to recover the original information. On this basis, a metasurface wireless communication system based on BASK and BPSK is constructed to transmit digital image information in a real-world environment. In the experiment, the image is first represented by a sequence of 0 bit and 1 bit, corresponding to the operational state sequence of the metasurface used for transmitting information. The field programmable gate array (FPGA) is then used to generate signals with high and low voltage levels in real time according to the sequence of working states of the metasurface, and to modulate the carrier signal irradiated onto the metasurface. Therefore, the signal is converted into a modulated signal and received by the antenna. Finally, the signal is demodulated by the universal software radio peripheral (USRP) and transmitted to the terminal equipment, yielding the constellation diagrams and enabling the recovering of the images. The image information recovered under both modulation schemes verifies that the system can achieve real-time modulation and transmission of digital information. The proposed metasurface and the design method may be used in many fields, such as satellite communications and digital broadcasting.
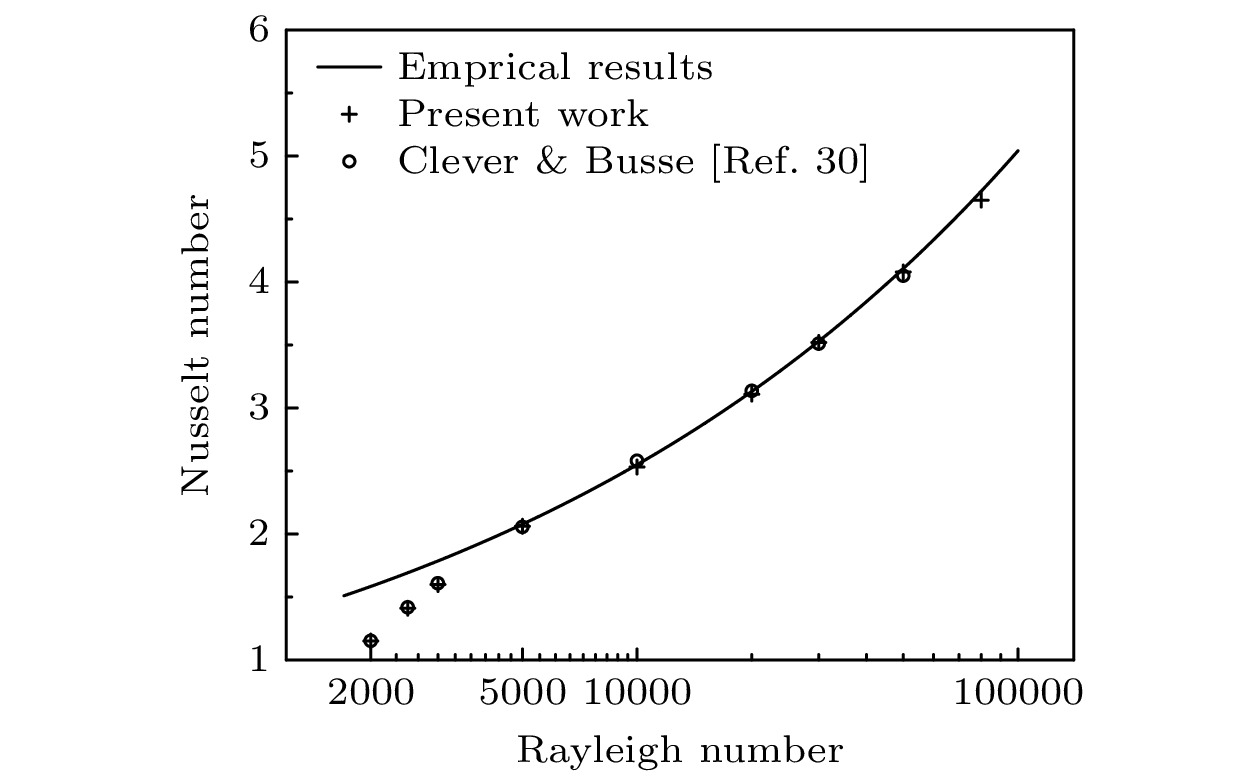
2025, 74 (10): 108102.
doi: 10.7498/aps.74.20241702
Abstract +
The solidification process of alloy ingot is often accompanied by the phenomena of free dendrites growing and colliding with each other while moving, which has a non-negligible influence on the temperature field, flow field, solute field and microstructure of the ingot, and it is one of the key issues in the study of ingot solidification organization formation. The cellular automata-lattice Boltzmann (CA-LB) coupling model has been developed rapidly in recent years in dealing with the moving dendrites, which can not only maintain the morphology of the moving dendrites well, but also calculate the mutual collisions between the dendrites reasonably. In this work, the cell-automata-lattice Boltzmann model for simulating the growth of free dendrites is improved. Alternating direction implicit iteration method is used to solve the differential heat conduction equation, and the simulation parameters are not limited by stability conditions in this method. In this research, the accuracy of the flow-solid coupling of the model is verified by taking the flow around a circular cylinder for example, and the temperature field of the model is well coupled under the natural convection condition. Finally, the solidification process of Fe-0.34%C alloy ingots with or without equiaxed grain movement is simulated using this model. The simulation results show that the movement of equiaxed grains increases the contact probability with the neighboring dendrites, which leads to a more uniform grain size in the ingot; the movement of dendrites also changes the solute distribution in the center of the melt, especially increasing the size and range of the hot-top segregation; the movement of equiaxed grains is impeded by the columnar crystals, and therefore the CET region is not much affected by the movement of dendrites.
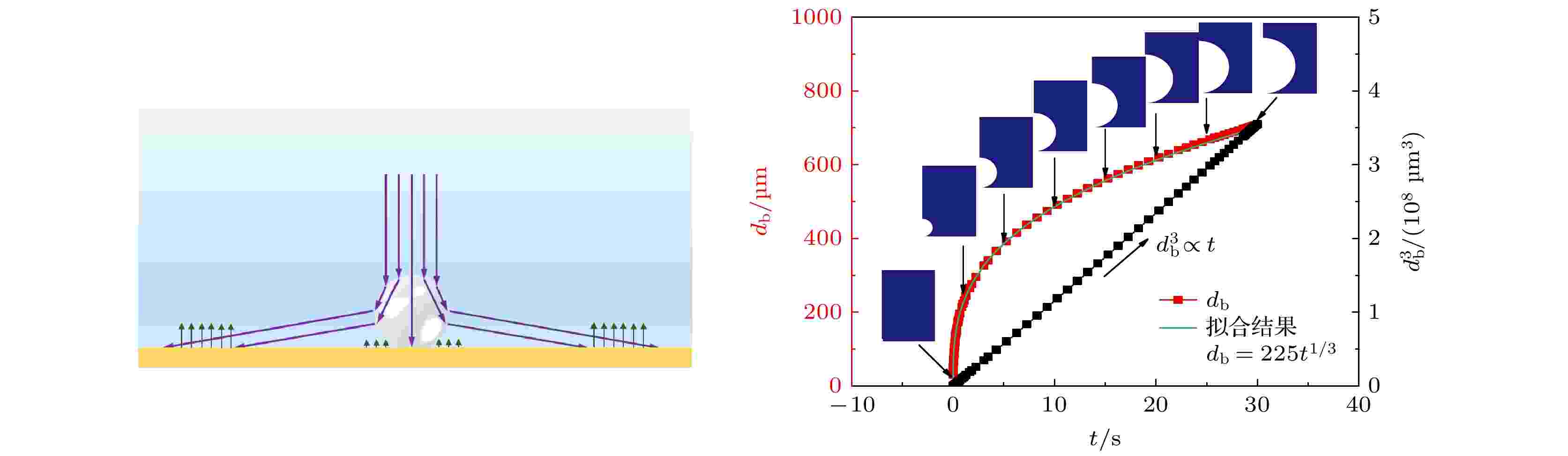
2025, 74 (10): 108201.
doi: 10.7498/aps.74.20250014
Abstract +
In order to enhance the efficiency of large-scale water decomposition, it is important to understand the oxygen bubble evolution on the electrode surface. In this work, a numerical model for the growth of oxygen bubbles on the electrode surface is proposed based on the dissolved oxygen flux at the bubble boundary, and the mechanisms of the reaction area and current during the bubble growth are investigated. The results show that the bubble diameters calculated from the oxygen flux at the bubble boundary are in good agreement with the diameters of the bubbles growing in the control phase of the chemical reaction. As the reaction region increases, the transition time from the diffusion-controlled stage to the chemical reaction-controlled stage becomes longer during the bubble growth. The concentration maximum value on the microelectrode surface is significantly higher than that on the large electrode surface, which leads to a steeper concentration gradient between the microelectrode surface and the bubble surface. As the current increases, the bubble growth rate increases and the time coefficient decreases faster. The bubble diameter at a current of 0.06 mA accords well with the bubble diameter at a current of 0.1 mA in the photoelectrochemical water splitting experiments. This is because the scattering of light by the growing bubbles leads to a decrease in the current density at the bottom of the bubble.
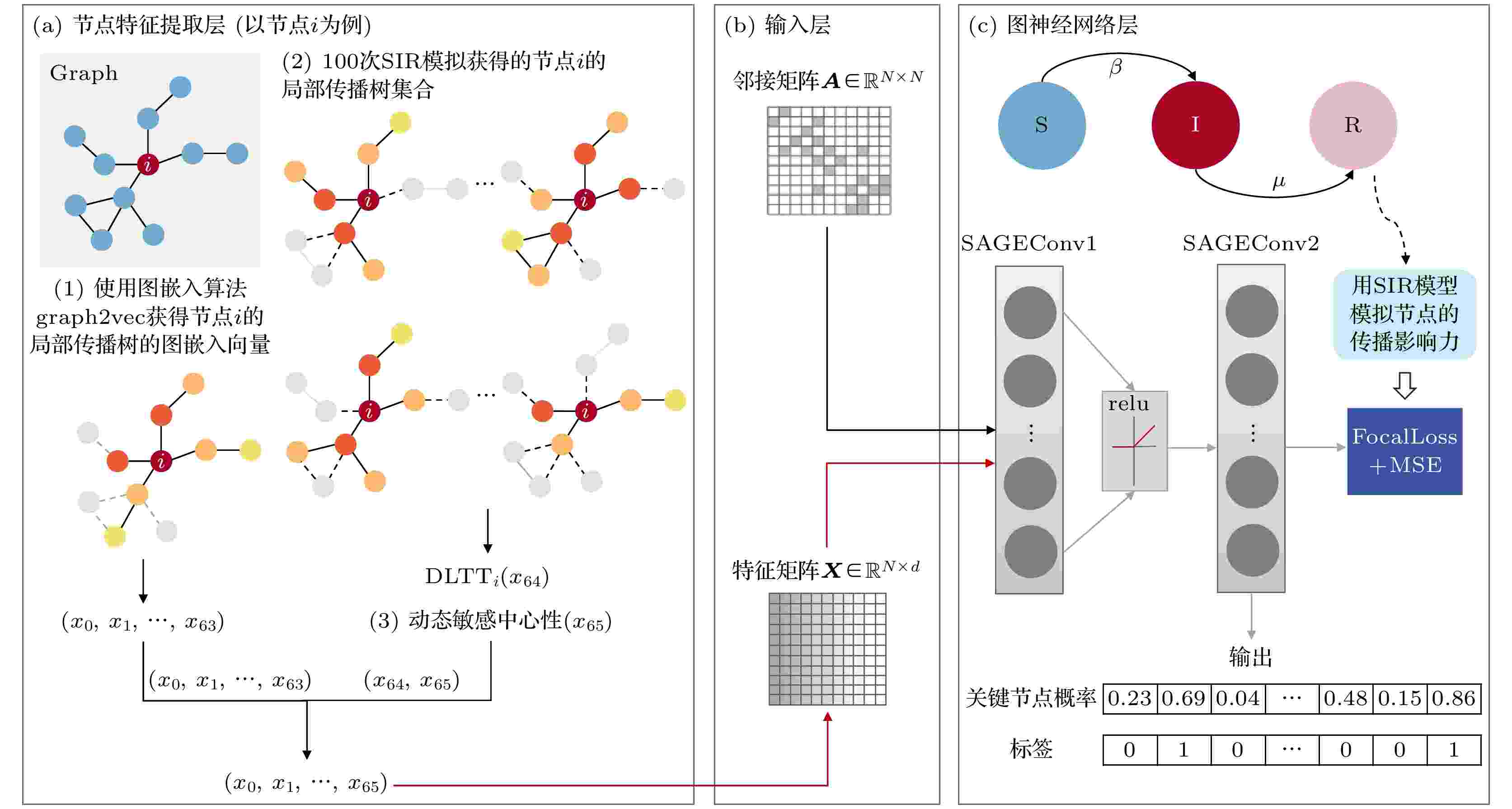
2025, 74 (10): 108901.
doi: 10.7498/aps.74.20250179
Abstract +
Identifying the most influential nodes in the spreading process in complex networks is crucial in many applications, such as accelerating the diffusion of information and suppressing the spread of viruses or rumors. Existing methods of identifying influential spreaders have their limitations. Specifically speaking, classical network centrality methods rely solely on local or global topology to predict node influence; traditional machine learning and deep learning methods are not suitable for graph-structured data; existing graph neural network-based methods neglect the dynamic characteristics of the propagation process itself. Researchers have pointed out that the spreading influence of nodes not only depends on their structural location, but is also significantly influenced by the dynamics of the spreading process itself. In this work, we propose a propagation dynamics graph neural network (PDGNN) that integrates the dynamic features of the propagation process and the structural features of nodes to identify influential nodes. Specifically speaking, based on the susceptible-infected-recovered (SIR) propagation model, the dynamic infection features and potential infection capacity of nodes are extracted from the epidemic spreading process. Then a high-dimensional feature vector of each node consisting of the embedding and degree of its local transmission tree, as well as its dynamics-sensitive centrality is constructed and used as the input to the graph neural network. To deal with the problem of imbalanced numbers between critical nodes and non-critical nodes in training the model and optimizing the output, an optimized loss function is designed, which combines focal loss with mean squared error. Experimental results in two synthetic networks and seven real-world networks show that the PDGNN outperforms classical centrality methods, traditional machine learning and deep learning-based methods, and existing graph neural network-based methods in identifying influential nodes in the spreading process in complex networks. The performance of PDGNN is robust when the infection rate and the size of the training set change. In a wide range of infection rates, the proposed PDGNN can accurately identify influential spreaders. Despite the fact that the training set accounts for 30% of the total dataset, the PDGNN has the smallest inaccuracy in all nine studied networks.